The Diels-Alder Reaction
Introduction to the Diels-Alder Reaction
In this tutorial, I want to talk about probably one of the most important organic reactions of the 20th century—the Diels-Alder reaction. And trust me, that’s not just my opinion. The importance of the Diels-Alder reaction has been recognized by some of the greatest minds in organic chemistry, including Nobel laureates Robert Woodward and Elias Corey.
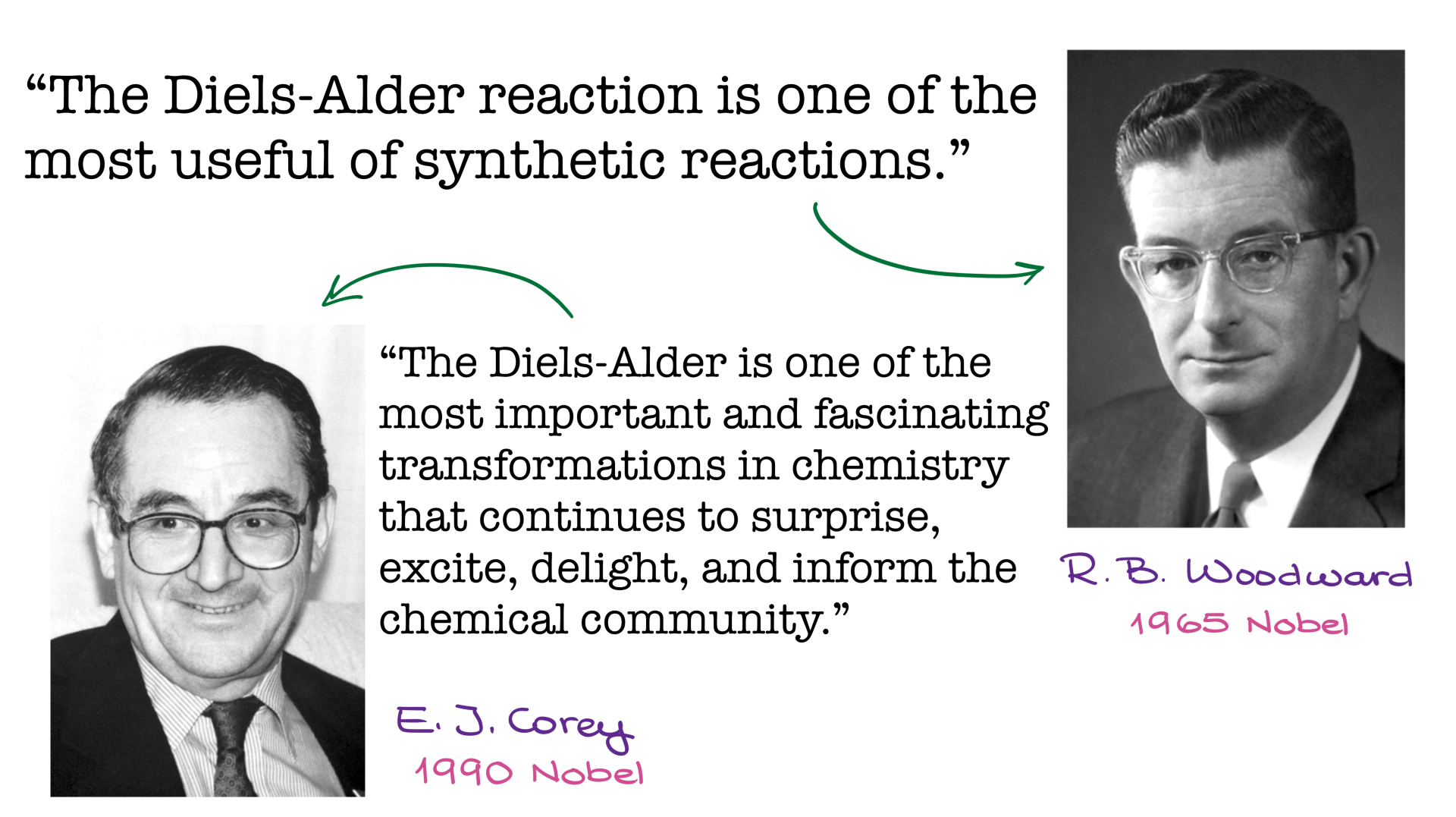
What is the Diels-Alder Reaction?
The Diels-Alder reaction was first reported by Otto Diels and his doctoral student Kurt Alder in their 1928 paper. It quickly captured the hearts and minds of the organic chemistry community thanks to its versatility and unmatched ability to form cyclohexenes loaded with substituents.
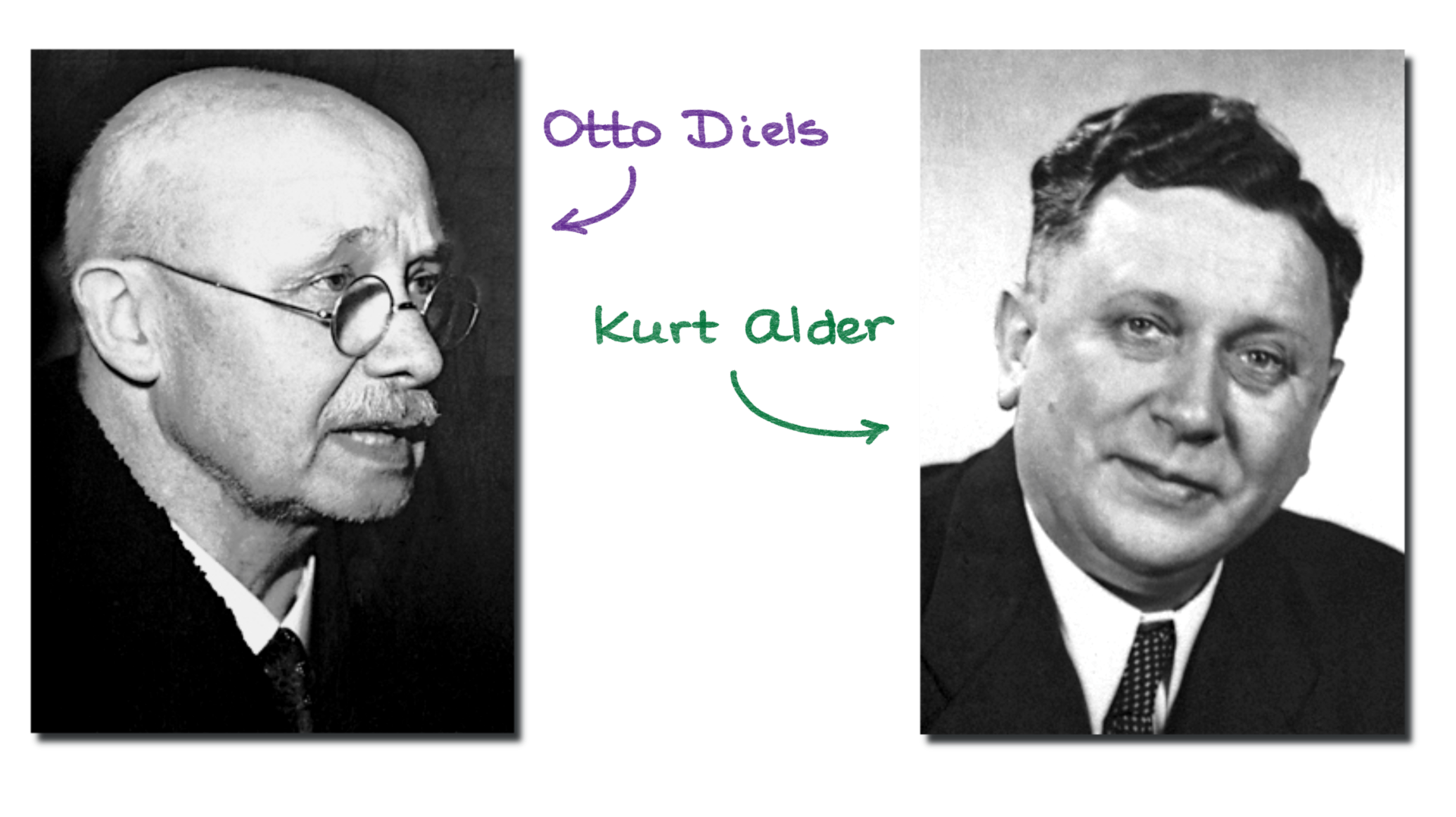
So what exactly is the Diels-Alder reaction? Well, if we take a diene—like butadiene—and react it with another alkene or alkyne—like ethylene—we get a new six-membered ring.
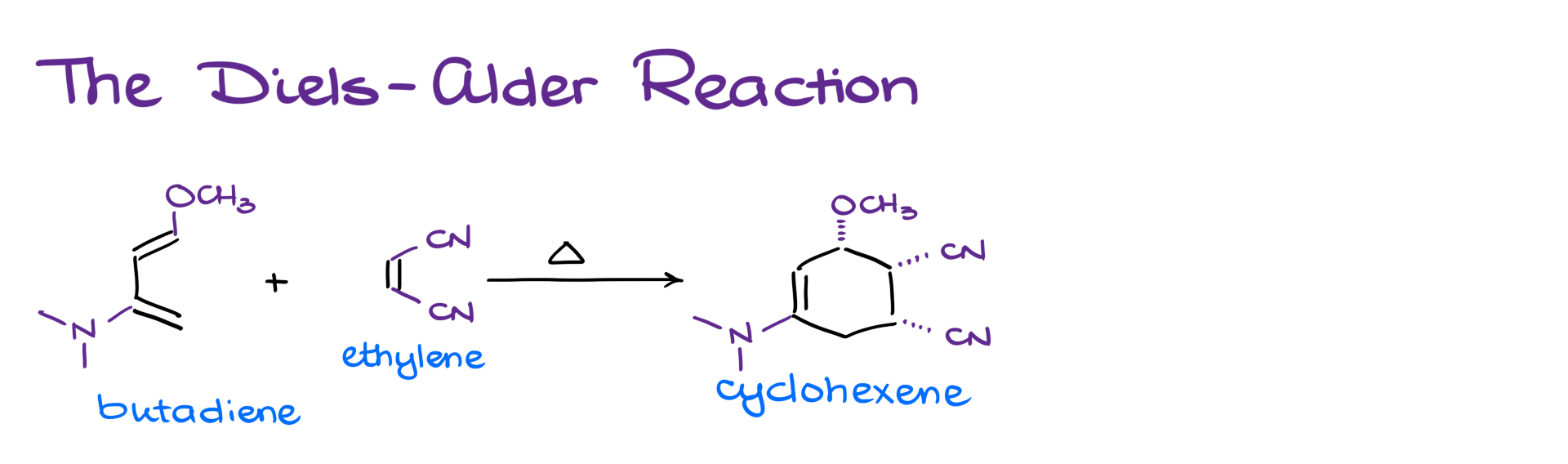
Of course, when I show the reaction that way, it might not seem very exciting. But once I dress up my molecules with different substituents, suddenly, I’ve got a very complex product—something that would be really hard to make using other synthetic methods.
The Mechanism of the Diels-Alder Reaction
Now, do you want to know the best part of the Diels-Alder reaction? It’s the mechanism, of course! And do you want to know the best part about the mechanism? It doesn’t matter how you show it—it’s going to be correct!
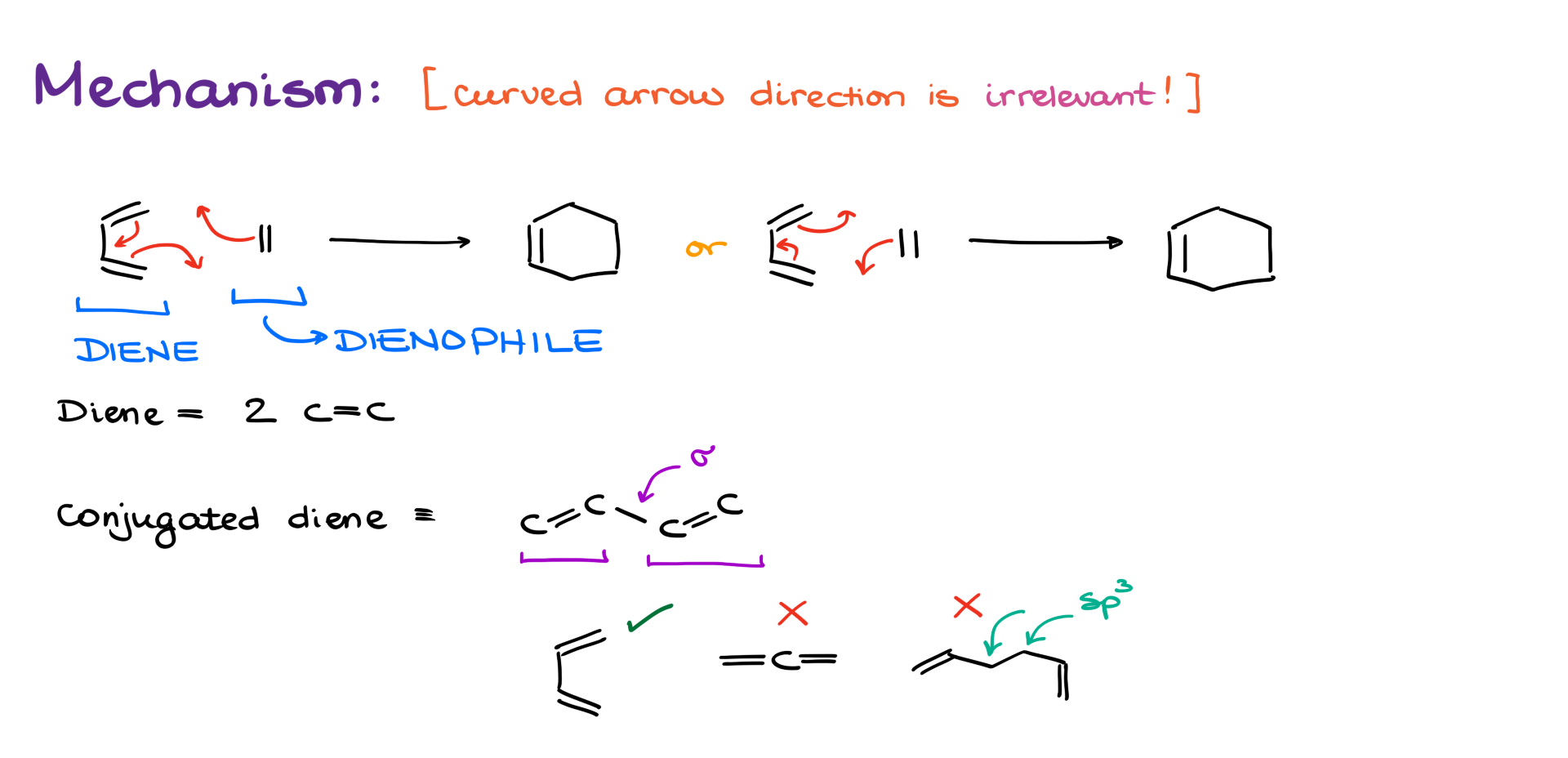
And no, I didn’t fall headfirst off a mountain during my last hike—I promise I’m not delusional. Let me explain.
Up until now, we’ve always told you that the nucleophile donates electrons to the electrophile in any organic reaction. So, we start our curved arrow at the nucleophile and point it toward the electrophile. That’s fine when we have electrophiles and nucleophiles. But in the Diels-Alder reaction, there are none. Still, electrons move! (Sort of.) So we still need to show electron movement with curved arrows. But here’s the fun part: it doesn’t matter if you draw them clockwise or counterclockwise—both are correct!
How exactly this works and why the molecular orbitals interact the way they do is beyond the scope of this tutorial, so I’m not going to bore you with those details now.
Diene and Dienophile
Before we move on to examples, I want to go over a couple of terms we’ll be using. First, the “diene.” Pretty straightforward—it’s a molecule with two carbon-carbon double bonds. But for the Diels-Alder reaction, we specifically need a conjugated diene. That means the two double bonds are separated by just a single bond—no interruptions—so they can participate in the reaction.
Next up is the “dienophile,” which literally means “diene lover.” It can be either a double or a triple bond. Basically, the dienophile is the thing that reacts with the diene to form our product.
Alright, ready to move on?
How to Keep Track of Atoms in the Diels-Alder Reaction
Now that we understand how the reaction works, it’s important to keep track of the atoms from the starting materials all the way to the product. Since we’ll be rotating molecules and shifting them around in space, the final product won’t always look much like the reactants—and it’s easy to lose track of which group ends up where.
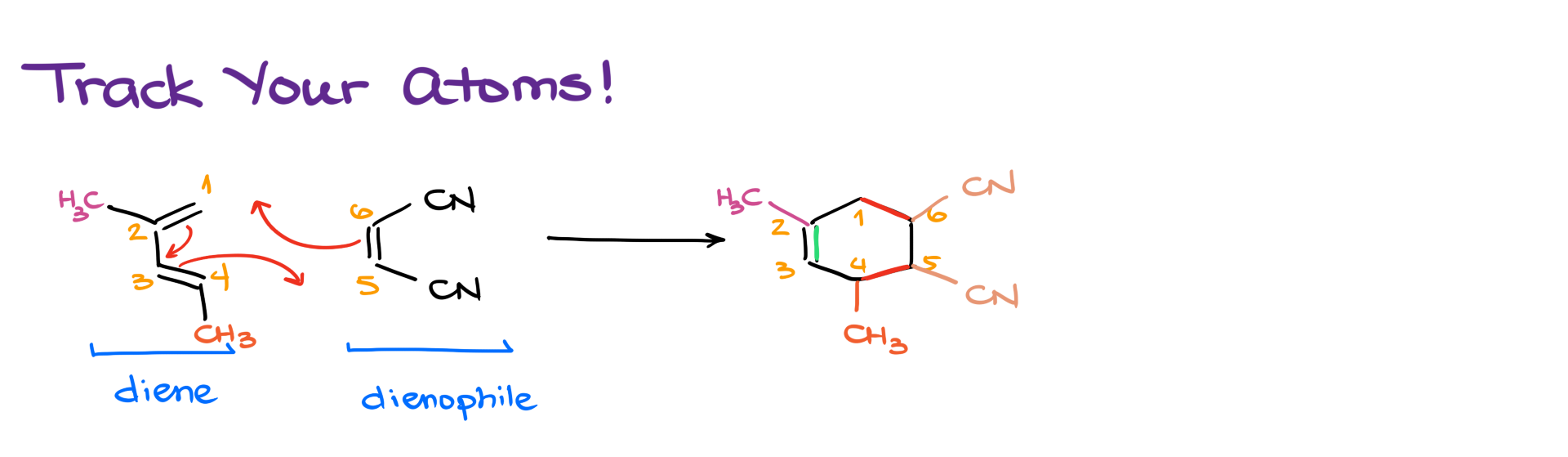
So here’s an easy way to keep everything straight.
Start by positioning your diene on the left in a “C” shape. Then, number the atoms of the diene from 1 to 4—I like to start from the top. Draw your dienophile on the right and number the atoms in the double or triple bond as 5 and 6.
Next, sketch the basic skeleton—or “stem”—of the final product. Number it accordingly. Finally, add all your substituents to their correct positions.
And that’s it! This method helps you avoid misplacing groups and losing points on your exam for something totally avoidable.
Examples of the Diels-Alder Reaction
Alright, are we ready for some examples?
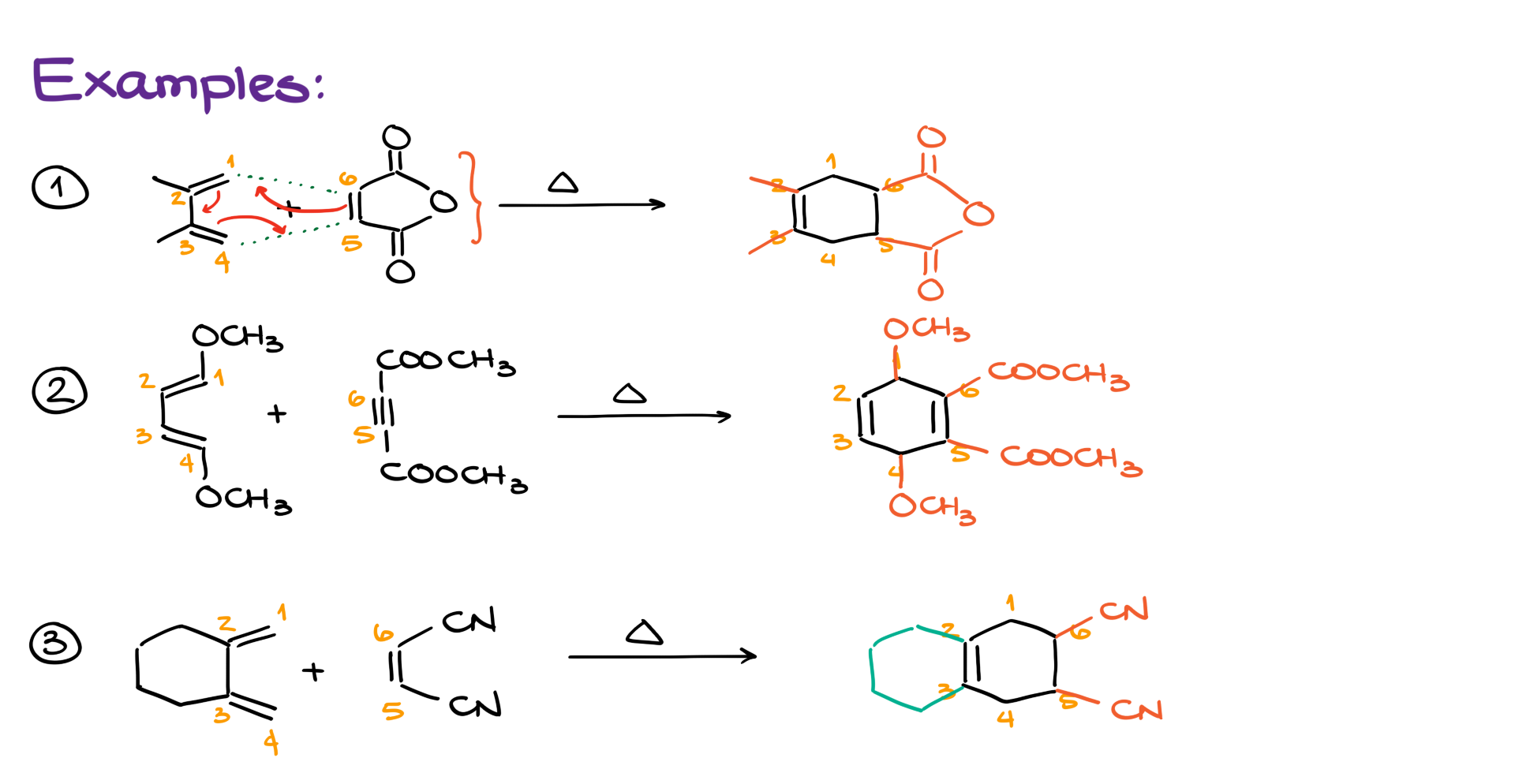
s-Cis vs s-Trans Conformations of Dienes
Hello everyone, Victor here—your organic chemistry tutor. In this tutorial, I want to continue our conversation about the Diels-Alder reaction, this time focusing on the requirements we have for our dienes.
Conjugated Dienes
As I already mentioned earlier, not every diene works for this reaction. The first requirement is that the diene must be conjugated. In other words, we can only use dienes that have two carbon-carbon double bonds adjacent to each other, separated by a single σ-bond.
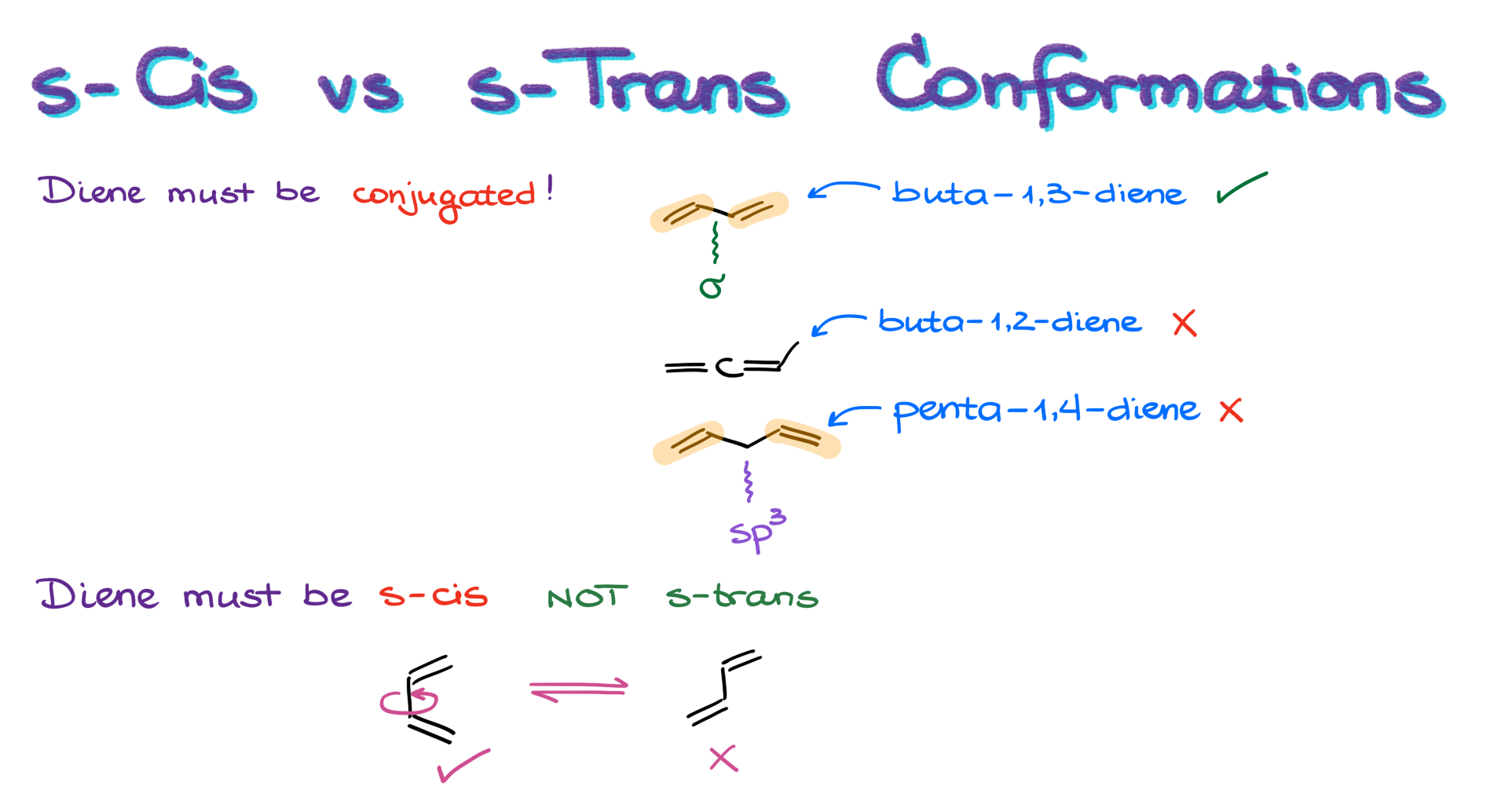
For example, buta-1,3-diene is a conjugated diene. But buta-1,2-diene isn’t—it’s not conjugated. Same with penta-1,4-diene; it doesn’t qualify because the two double bonds are separated by an sp³-hybridized atom, which breaks the conjugation.
s-Cis vs s-Trans Conformations
Now, here comes the part that trips up a lot of students: the diene needs to be in—or at least able to assume—the s-cis conformation. So, what’s the difference between s-cis and s-trans?
This has nothing to do with the stereochemistry of the double bonds themselves. What we’re talking about is the overall orientation of the diene. If the two double bonds form a C-shape, that’s an s-cis conformation. If they form more of a stretched-out zig-zag shape, that’s s-trans.
For the Diels-Alder reaction to occur, the diene needs to either already be in s-cis form or be able to rotate into it. That rotation happens around the single σ-bond between the double bonds. If it’s tough to visualize, I highly recommend grabbing your molecular model kit and making a simple butadiene. Try rotating it around the central single bond—it’ll help you understand what’s going on.
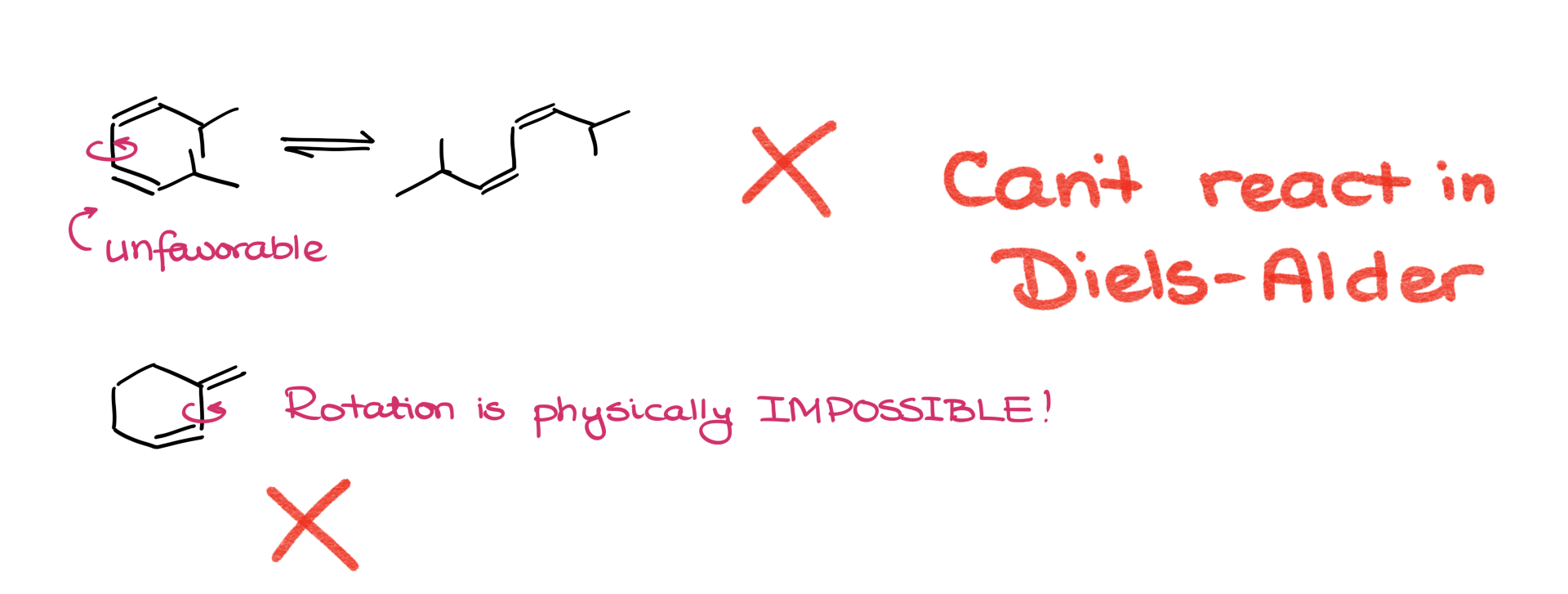
Now here’s the catch—not every diene can rotate into s-cis. Sometimes there are steric hindrances that prevent the molecule from adopting that conformation. Or maybe the structure is just physically locked in place. In those cases, the molecule simply can’t participate in the Diels-Alder reaction.
Converting a Molecule from s-Trans into s-Cis Conformation
Most of the time, your diene will be given in the s-trans conformation, and it’s up to you to convert it into the corresponding s-cis form—assuming the molecule allows for it. And this is where students often struggle. Let’s be real—not everyone’s great at 3D visualization, and rotating molecules in your head can feel impossible. But as I always say, if you can’t do it in your head, do it on paper!
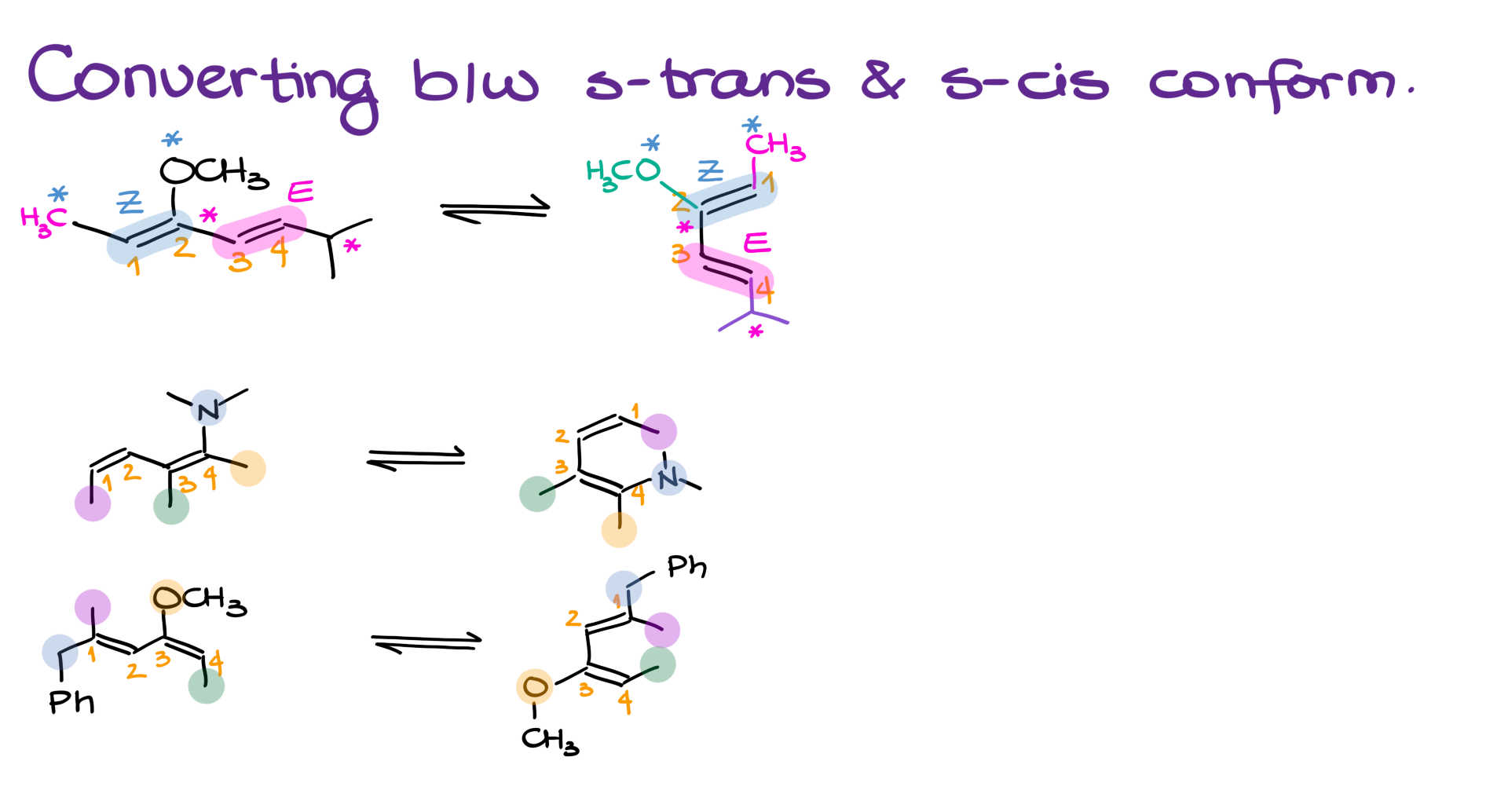
Let me show you what I mean. Suppose we have (3Z,4E)-3-methoxy-6-methylhepta-2,4-diene, and it’s drawn in the typical zig-zag, s-trans conformation. To convert it into s-cis, here’s what I would do:
First, I’d number the diene atoms 1 through 4.
Next, I’d draw the base of the s-cis conformation in that classic “C” shape.
Then, I’d add the substituents to my diene, making sure I’m respecting the stereochemistry of each double bond.
The trickiest part here is not accidentally flipping the stereoconfiguration of your double bonds! When you move molecules around in space, everything moves. So if something was pointing in one direction in the original drawing, it doesn’t have to point the same way after you reorient the molecule. Maybe it still does, maybe it doesn’t. You have to stop and analyze the structure as you go—don’t just copy what you had before without thinking.
Regioselectivity of the Diels-Alder Reaction
Let’s take a look at the following example.
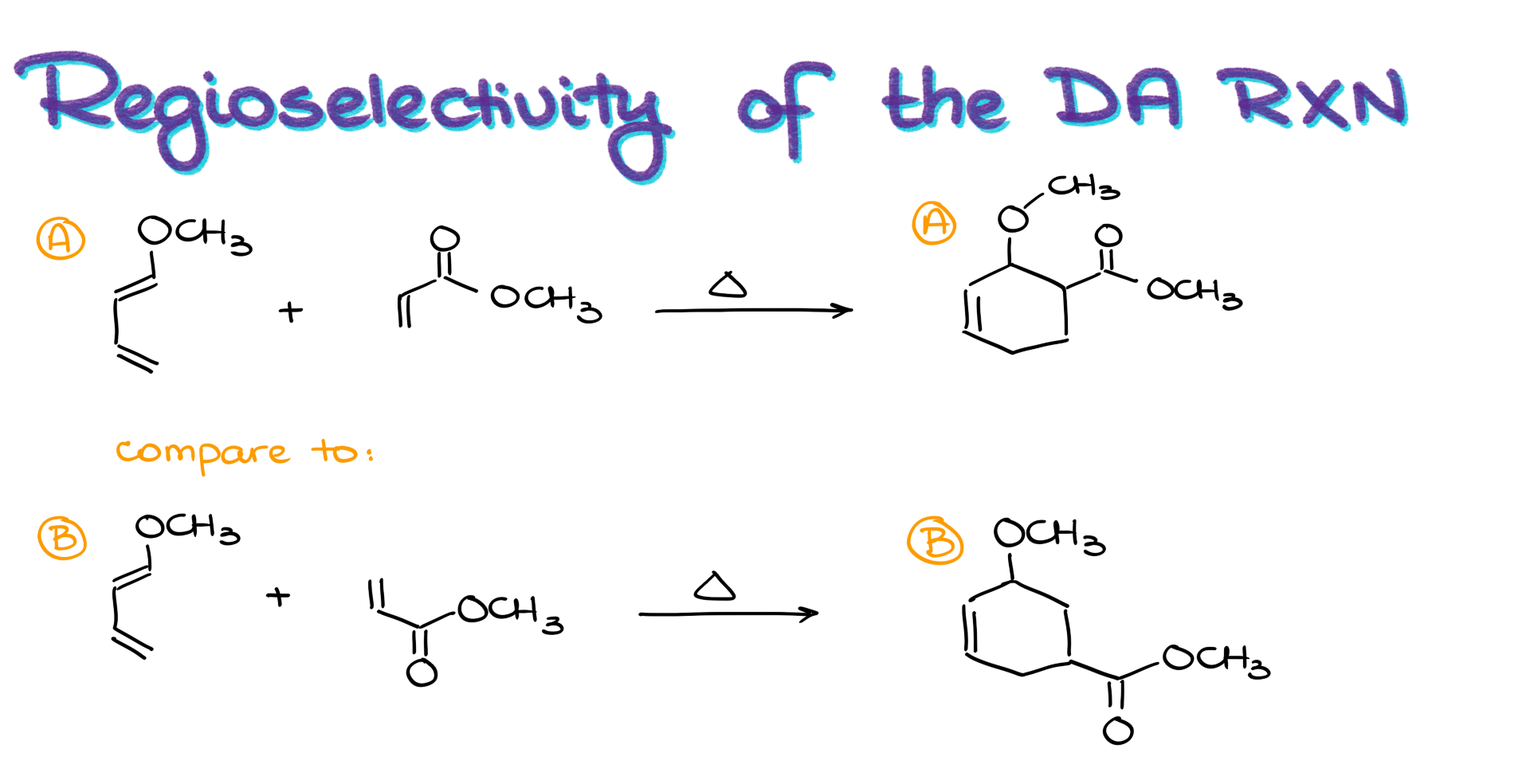
In this case, the molecules can react in two different ways, leading to two different constitutional isomers as products. So the big question is—will both of these products form in equal amounts, or is one going to be the major product?
This is where two common traps often trip students up.
Trap #1: A lot of students forget that molecules don’t care how we draw them. As a result, they fall into the trap of drawing the product based solely on how the starting materials were presented.
I’d be willing to bet that if I showed this reaction on a test using the setup from the first combination, most students would give me the first product. And if I flipped it to the second version, the majority would choose the second product—without giving it a second thought. Experienced instructors know this, and they’ll try to catch you with it on the exam.
Trap #2: Students often overemphasize steric hindrance and the relative stability of the products.
Now don’t get me wrong—sterics matter. And the thermodynamic stability of your products matters too. But you need to know when those factors are actually important. The thermodynamic stability of your products only plays a role when the reaction is reversible and is allowed to reach equilibrium.
Is the Diels-Alder reaction reversible? Technically, yes—it is an equilibrium. But it’s a very slow one. And under normal reaction conditions, we’ll never actually reach that equilibrium. That’s why the Diels-Alder reaction is considered a kinetically controlled reaction. In other words, the product that forms the fastest ends up being the major product.
So, how do we figure out which product forms faster in this case—and why?
The key lies in the polarization of the molecules due to resonance.
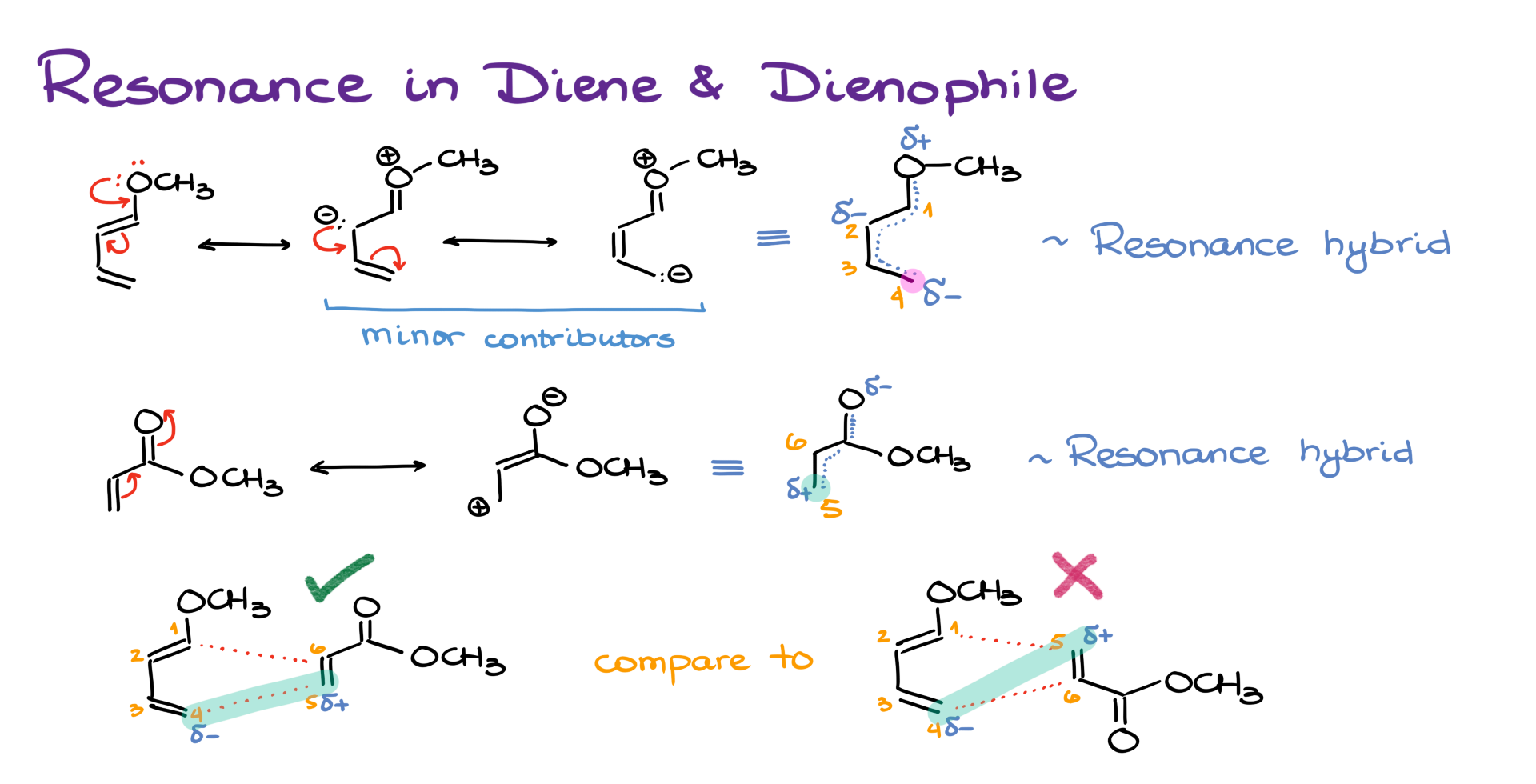
Let’s look at the diene first. It has an electron-donating group, and the oxygen in that group polarizes the diene. If I number the atoms 1 through 4, I’ll find that carbon #4 carries a partial negative charge as a result of resonance.
Now, looking at the dienophile, the carbonyl group polarizes the double bond. Through resonance, that gives us a partial positive charge on carbon #5.
So, when we bring these two molecules together, in the first combination, those partial charges are attracted to one another—making the transition state more favorable. But in the second arrangement, the dipoles don’t align well, leading to an awkward and less favorable transition state.
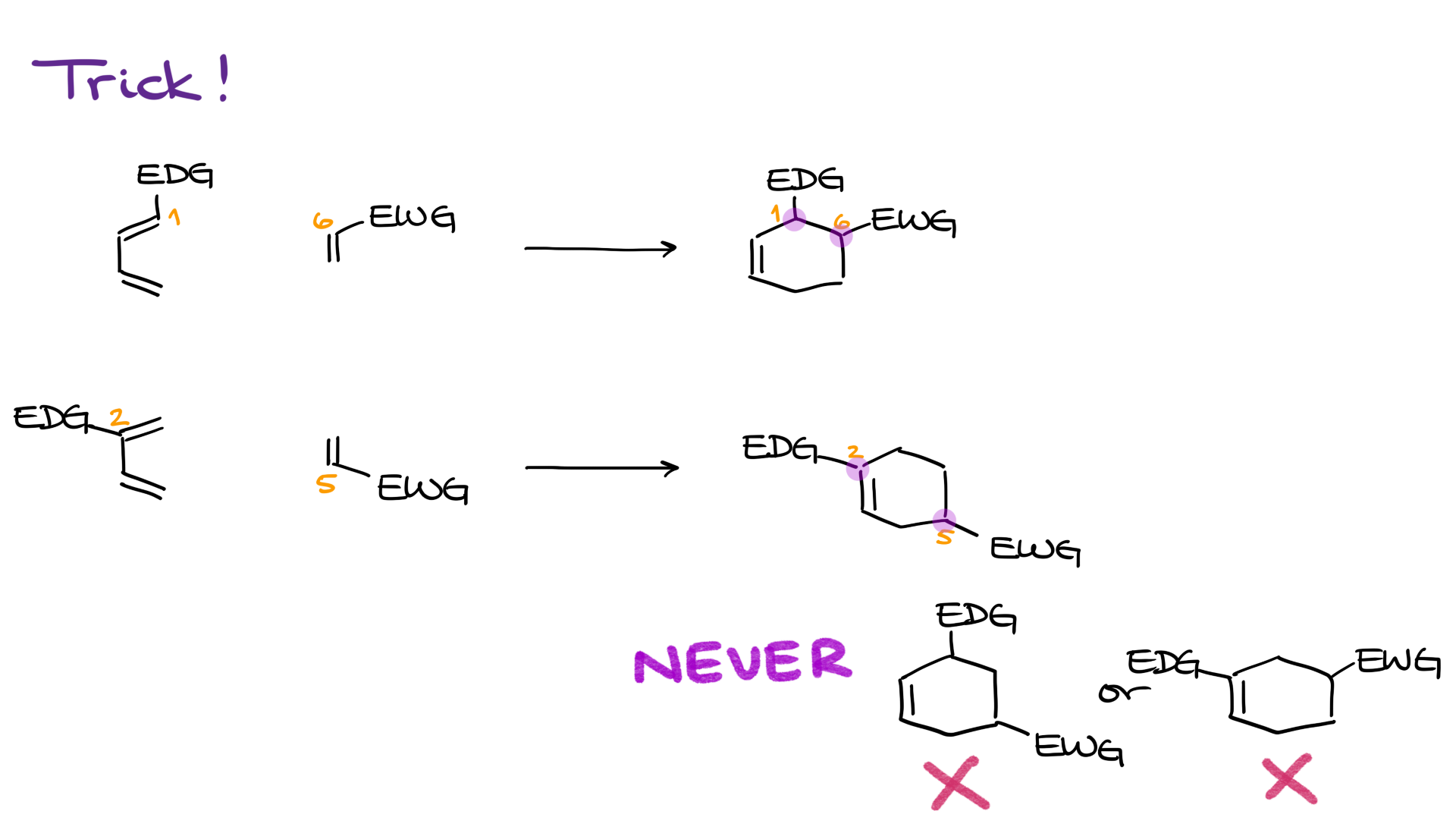
So the takeaway is this: if your diene and dienophile aren’t symmetrical, the very first thing you should do is check how each molecule is polarized using resonance structures. Only after that should you bring them together to see how the reaction would proceed.
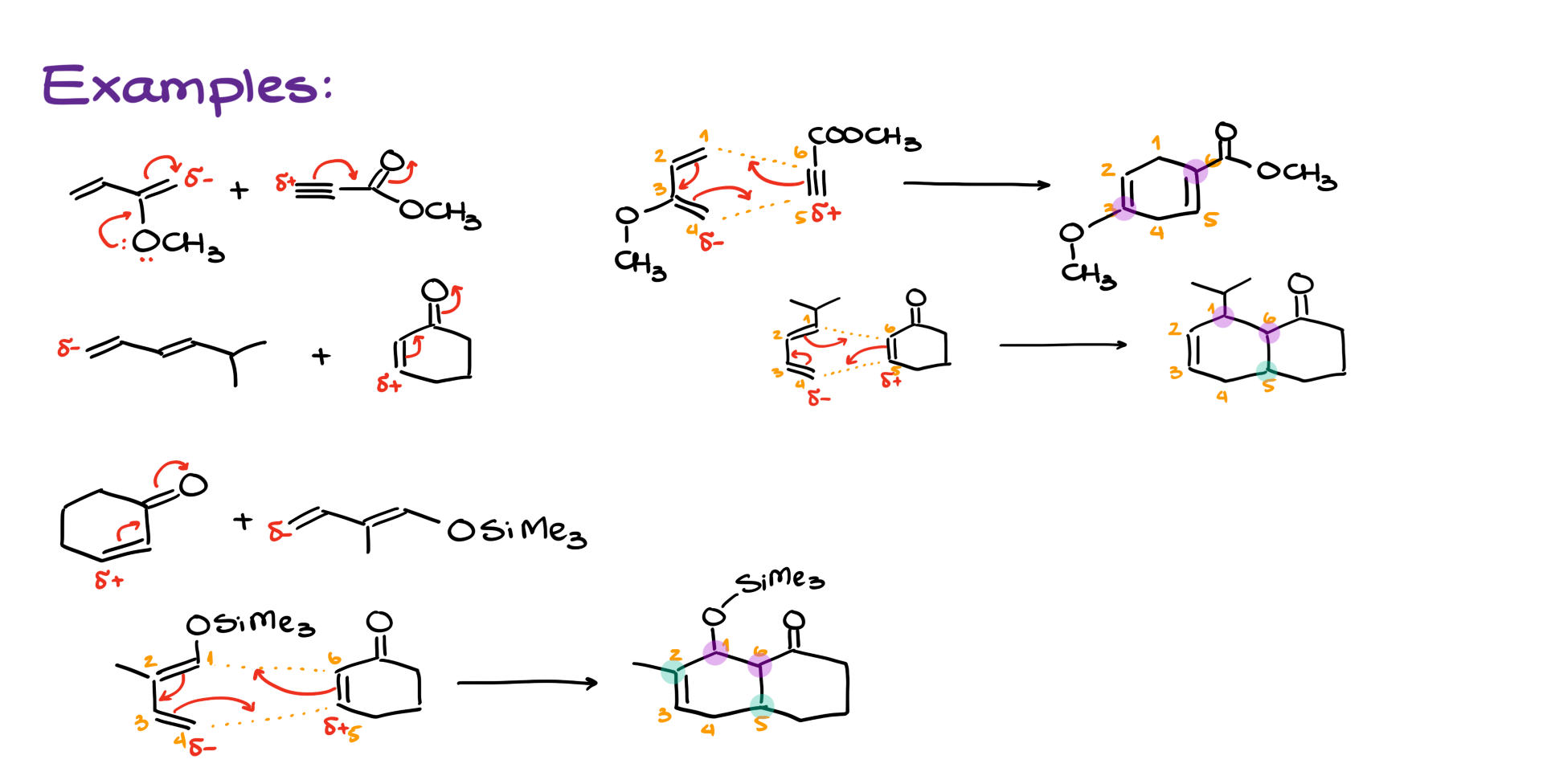
Try walking through these examples yourself. Pause the tutorial, work out the correct product based on resonance and polarization, and then compare your answers with mine.
Stereochemistry of the Diels-Alder Reaction
Let’s start with this reaction. We can form two possible products here. The one on the left is what we’ll call the exo-product, and the one on the right is the endo-product.
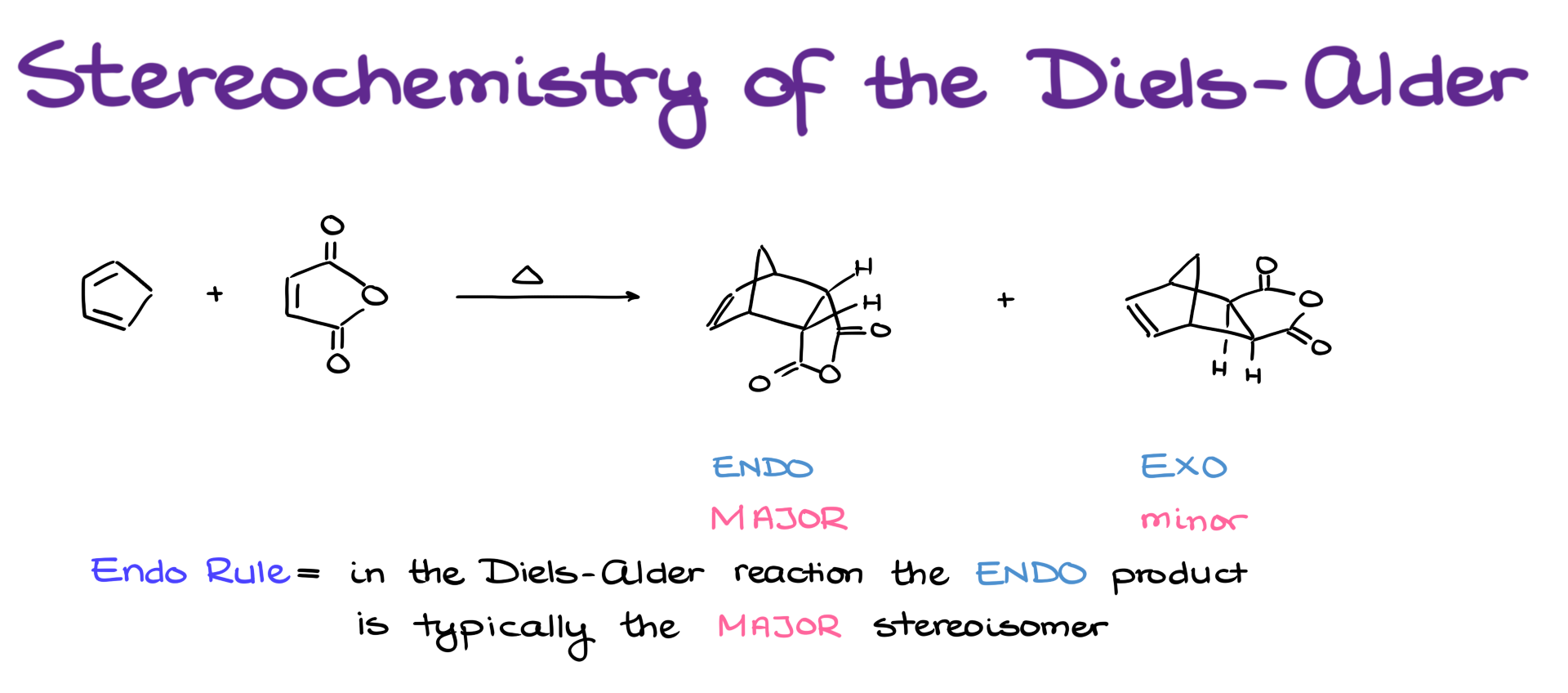
In terms of how we define them: the exo-product is where the dienophile is oriented away from the bridge (the one that has the double bond), while in the endo-product, the dienophile is oriented toward that bridge.
Now, the endo-product is typically the major product in the Diels-Alder reaction. This is known as the endo rule. And you might be wondering—why is that? The endo-product often looks less thermodynamically stable. And you’re absolutely right—it usually is less stable.
But the trick here is in the transition state. There’s a significant difference between the transition states that lead to the endo and exo products.
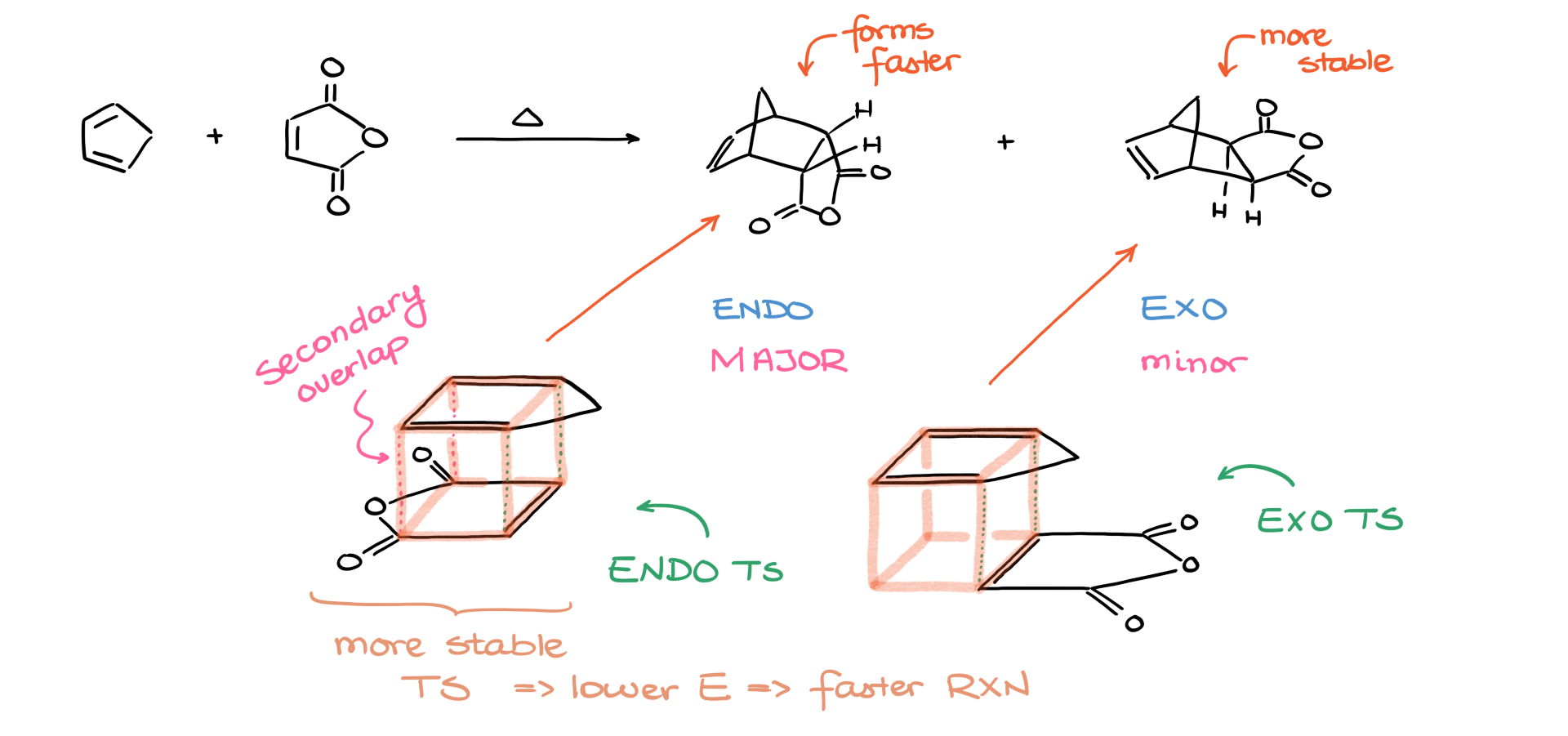
If we stack the diene and dienophile during the reaction, we can arrange them in two ways. One has the molecules stacked at an angle—this is the exo transition state. The other stacks them directly on top of each other—this is the endo transition state. What makes the endo arrangement special is the secondary orbital overlap between the diene and the dienophile. This extra overlap stabilizes the endo transition state, lowering its energy. And a lower-energy transition state means a lower activation energy, which means the reaction proceeds faster.
From a kinetics standpoint, the Diels-Alder reaction favors the kinetic product—the one that forms the fastest. And that’s the endo-product.
Now you might be thinking, “Isn’t the Diels-Alder reaction an equilibrium?” And yes—it is. But it’s an extremely slow equilibrium. Under normal conditions, we don’t give the reaction enough time to reach that equilibrium. So even though the exo-product might be more thermodynamically stable, it ends up being the minor product because we stop the reaction before equilibrium is achieved.
The Trick
Now, drawing the transition state for every Diels-Alder reaction—sometimes called the “cube method”—can be a bit tedious. And you’re probably not going to have time on a test to go through it every single time.
Luckily, I have a shortcut.
Before we go over the trick, let’s get a few definitions down. Take this diene here—it’s already in the s-cis conformation, so we don’t need to rotate anything. But if your diene isn’t already in the correct conformation, make sure you fix that first. (And if you need a reminder, check out my tutorial on the s-cis conformation.)
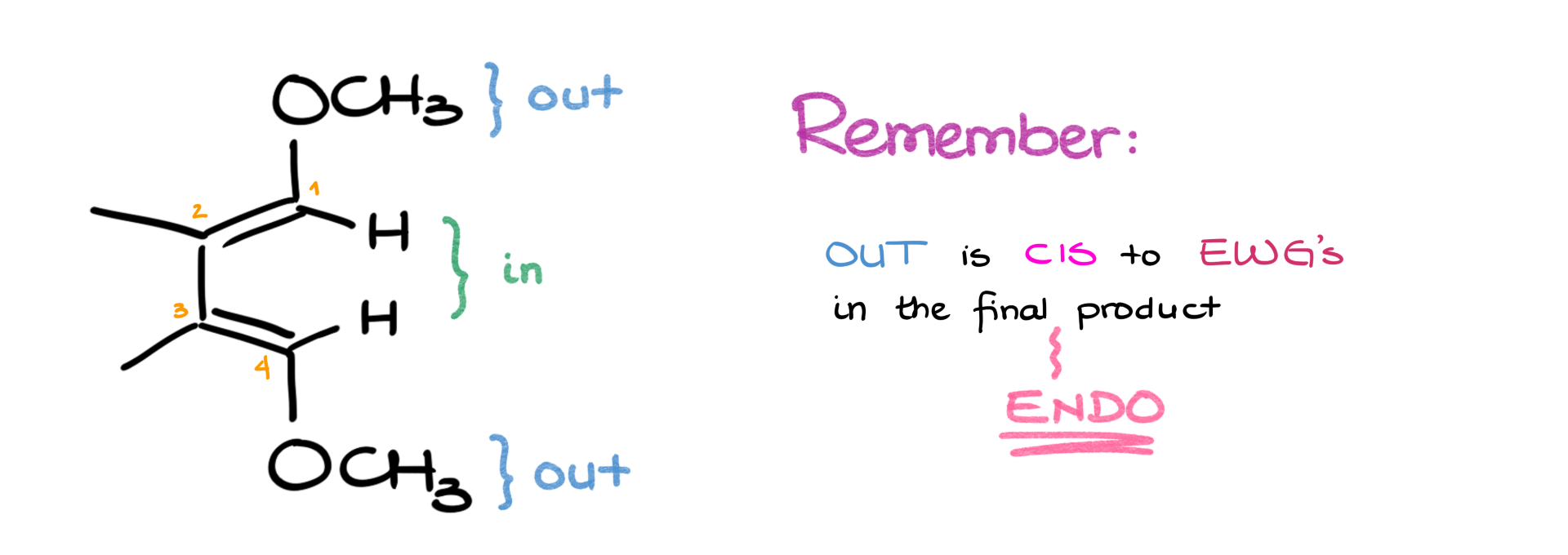
Back to our example. I’m going to number the atoms of the diene 1 through 4. When it comes to stereochemistry, we care most about the groups on atoms 1 and 4. We may have some groups on atoms 2 and 3, but those usually aren’t relevant here.
We’ll call the groups pointing inward—the ones facing into the reaction—as the “in-groups.” In this case, they’re hydrogens. The ones pointing away are the “out-groups”—the methoxy groups here.
Now here’s the part to remember: in the endo-product, the out-groups will be cis to the electron-withdrawing groups (EWG) on the dienophile, and the in-groups will be trans to those EWG’s.
So let’s use that to predict the product. Say I react this diene with a dienophile that has nitrile groups—those nitriles are my EWG’s. The methoxy groups on atoms 1 and 4 are my out-groups, and the hydrogens are my in-groups.
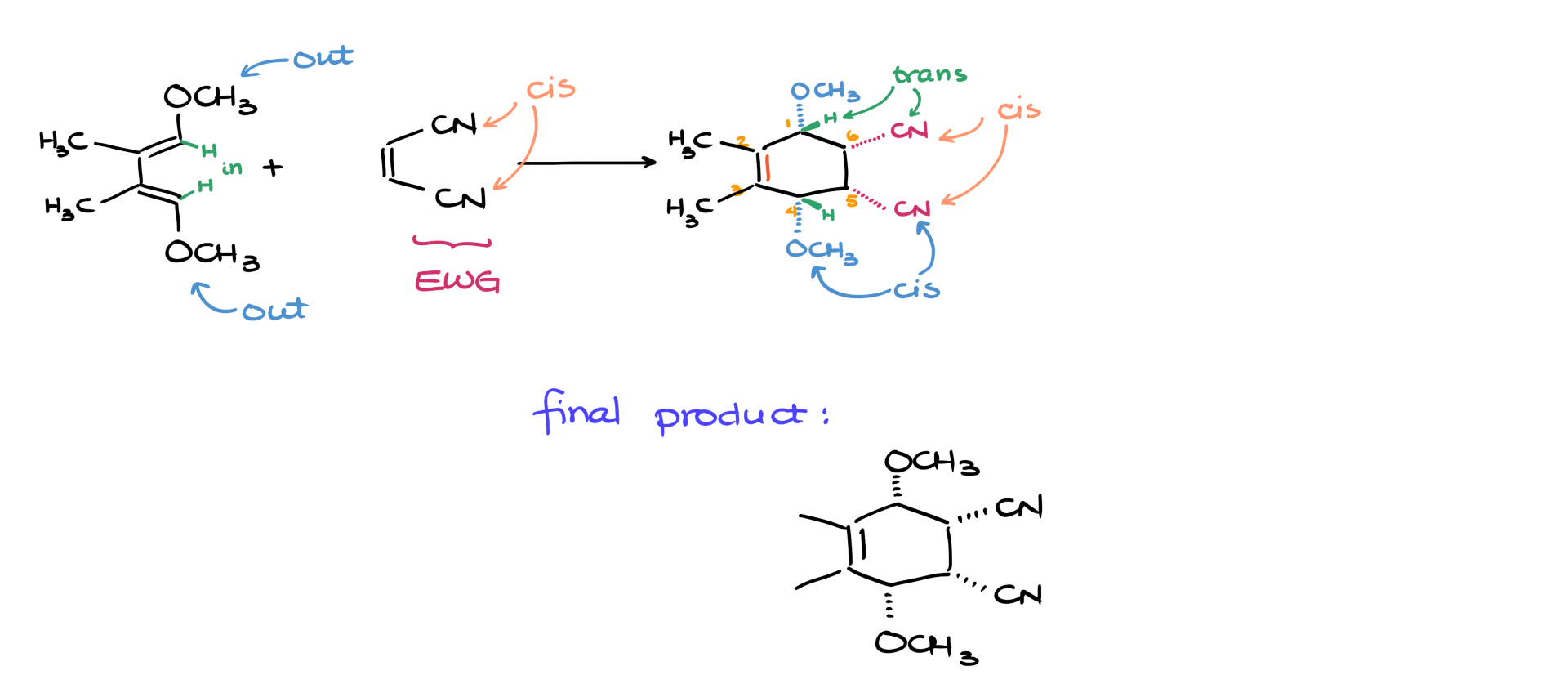
I’ll start by drawing the six-membered ring that’s the stem of my product. I’ll number the carbons and put a double bond between atoms 2 and 3. Next, I’ll add the nitriles to atoms 5 and 6—and since they were cis in the starting material, I’ll place them cis in the product, let’s say on dashes.
Now comes the key part. Since the out-groups must be cis to the EWG’s, I’ll also place the methoxy groups on dashes. The hydrogens—being trans—will go on wedges. Those can be implicit if you like. Finally, I’ll add the methyl groups on atoms 2 and 3, and that’s it! You’ve got your product.
This method might feel a little slow at first, but once you get used to it, you’ll fly through these steps in no time.
Stereochemistry of Bridged Bicycles
Of course, in real-world organic chemistry, we’re not going to stick with basic examples forever. Often, you’ll see reactions forming bridged bicyclic products, and drawing those with the correct stereochemistry can be tricky.
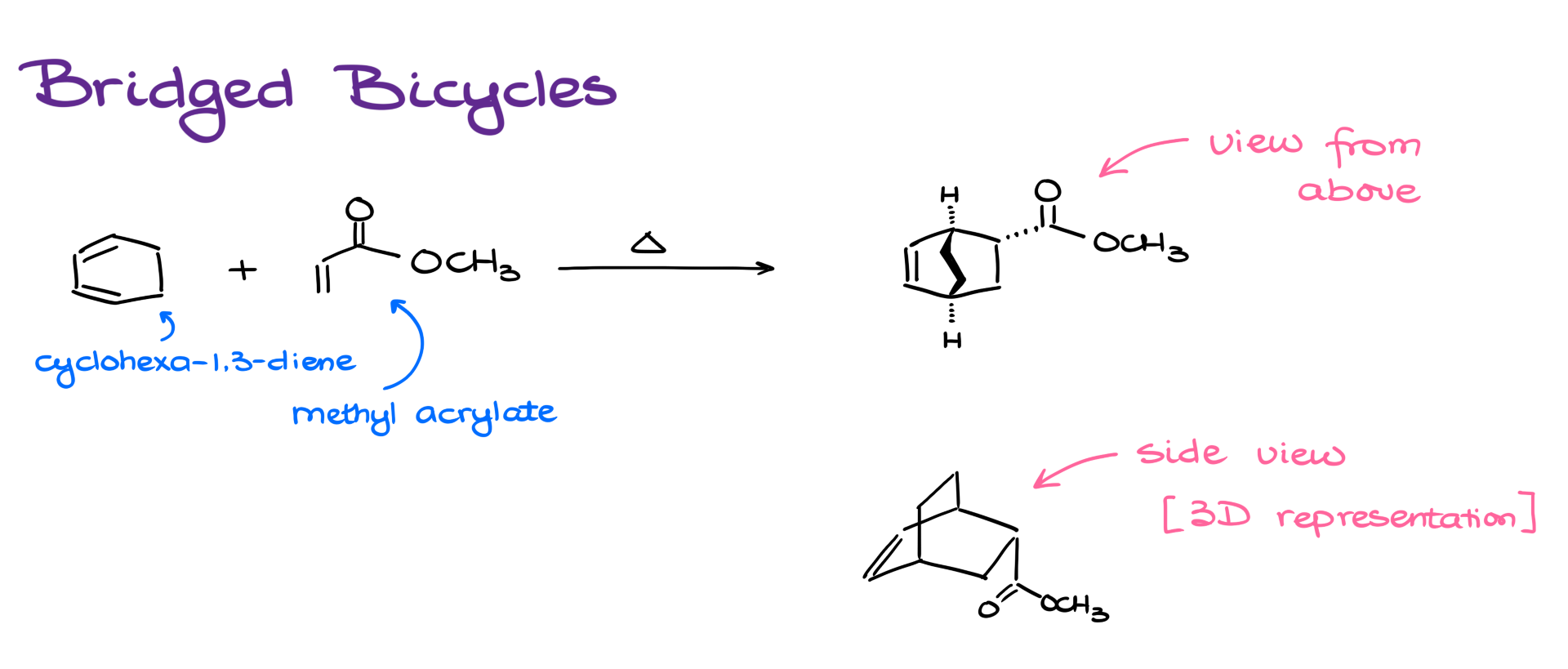
Let’s look at this reaction. I’m reacting cyclohexa-1,3-diene with methyl acrylate. So the diene is, well, the diene, and the dienophile is the ester. The complication here is that the in-groups on the diene are part of a continuous chain. That means they have to stay connected in the product too.
So, if I follow my usual steps to draw the product, I’ll get something that looks like this—a “view from above” drawing of the molecule. Notice how the bridge is facing toward us. And also notice—I didn’t disconnect any atoms that were previously connected. That bond stays intact.
Many instructors are perfectly fine with this top-down perspective. But some might require a full 3D representation—something like this side-view sketch of a bridged bicycle. This is a pretty standard way to represent these structures, so even if your professor doesn’t ask for it, it’s good to practice drawing them just in case.
Intramolecular Diels-Alder Reaction
In this section, I want to give you a step-by-step guide on how to deal with intramolecular Diels-Alder reactions. When it comes to regular Diels-Alder reactions, like the one I have in the scheme below, we typically don’t have any trouble predicting the product. But when it comes to the intramolecular versions of this reaction, things can get a little trickier.
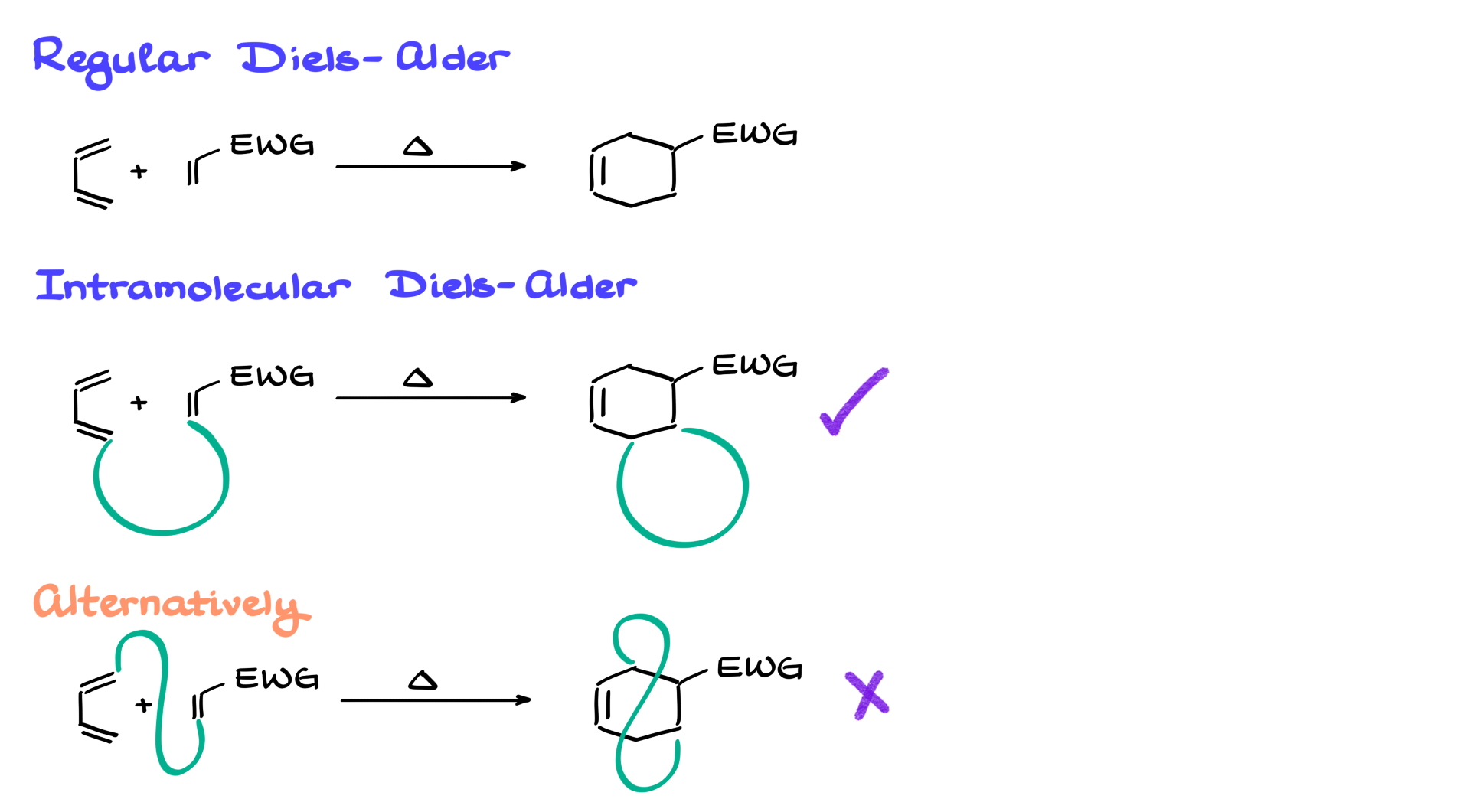
In the intramolecular version, the diene—the molecule on the left—and the dienophile are going to be connected to each other. I’ll show it very schematically as a semicircle. But because these two parts are connected, those same atoms are also going to be joined by a chain in our product as well, forming something like that. So, if a regular Diels-Alder reaction gives us one ring, the intramolecular Diels-Alder reaction gives us two rings right off the bat. Typically, we’ll be forming that extra ring off to the side of the molecule.
However, once in a blue moon—on a rare occasion—you might see a reaction that forms a strange-looking bridge going over the molecule, something like what I have in the second example. These cases are much more rare, so within the scope of your course, if you’re dealing with the intramolecular Diels-Alder reaction, it’ll usually be the first version and not the second.
Intramolecular Diels-Alder Reaction Example 1
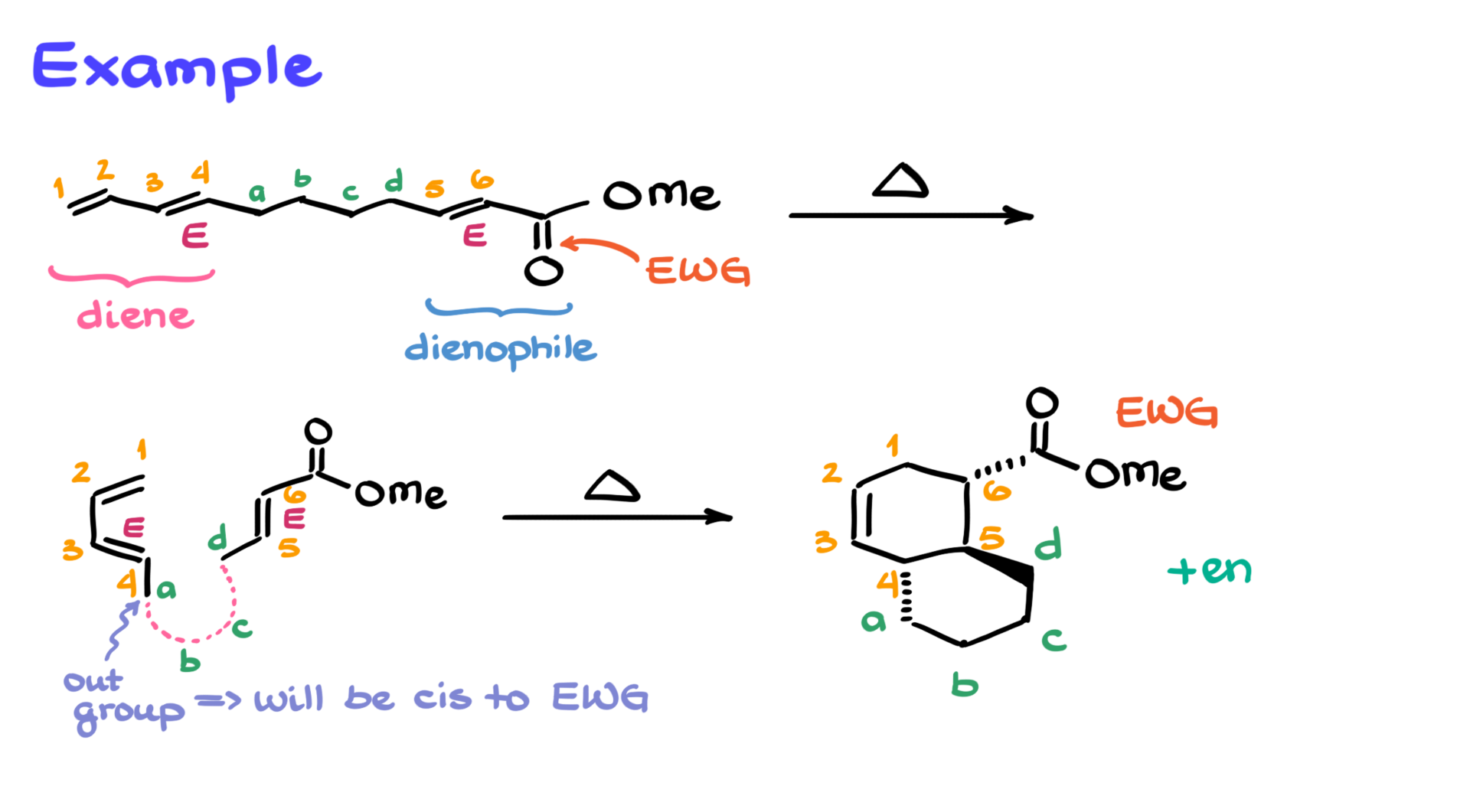
Now, molecules are virtually never given to you in a neat form where you just snap the pieces together, so there’s a bit of an algorithm to approach these kinds of questions. With that in mind, let’s look at my first example here. Trust me, this one’s probably one of the easiest intramolecular Diels-Alder reactions you’ll encounter.
Starting the analysis, I notice I have an electron-withdrawing group—a carbonyl—so that part of the molecule is most likely the dienophile. On the other side of the molecule, I see two double bonds right next to each other, so that’s going to be my diene.
To make my life easier, I’ll number the molecule—not all the way through, but in a way that helps predict the Diels-Alder product. I’ll number my diene carbons 1 through 4, and my dienophile carbons as 5 and 6. The middle portion, which connects them, I’ll label with letters A, B, C, and D because there are four carbons in that chain in this example.
I like doing it this way because it separates the two rings I’m forming. Carbons 1 through 4 and 5 and 6 will form the main six-membered ring in the Diels-Alder reaction, and atoms A through D will form the second ring.
Next, I’m going to redraw the diene, right away in the s-cis conformation—just how I need it for this reaction. I’ll also show carbons 5 and 6 of the dienophile nearby so it’s easier to visualize how the reaction is going to take place. I’m orienting everything so that atoms 4 and 5 are on the same side since they’re connected by the A-B-C-D chain.
With that setup, I’ll start adding the substituents. On carbon 6, I have the electron-withdrawing group, so I’ll draw that. Then comes atom A. It’s important to be very careful with stereochemistry so we don’t accidentally flip something.
In the original molecule, the double bond between atoms 3 and 4 was an E double bond (or trans if you prefer). So, when redrawing the molecule, I need to preserve that E stereochemistry. Likewise, atom D also needs to retain its E stereoconfiguration from the original structure.
Atoms B and C connect A and D, but I won’t draw them just yet to avoid cluttering the picture. I’ll just mark where they roughly go and forget about them for the moment.
Before drawing the product, I want to finalize the stereochemistry. Atom A is the out-group, which means in the final product it needs to be cis to the electron-withdrawing group on carbon 6. So, I’ll start by drawing the six-membered ring as the core of my product and number it 1 through 6, like I would in any Diels-Alder reaction. Then I’ll add the electron-withdrawing group to carbon 6—let’s say on a dash. It doesn’t matter whether you start with a dash or a wedge, but once you commit to one, the rest of the stereochemistry follows.
Since group A is cis to the electron-withdrawing group, I’ll also put it on a dash. Atom D, being trans, will go on a wedge. Now, I’ll sketch in where atoms B and C go, connect the bonds, and that gives me the final molecule. And because the attack can happen from either side, we’ll also get the corresponding enantiomer.
So now we have our final product with the correct stereochemistry. See? That wasn’t so bad.
Intramolecular Diels-Alder Example 2
Let’s try another one. How about this molecule?
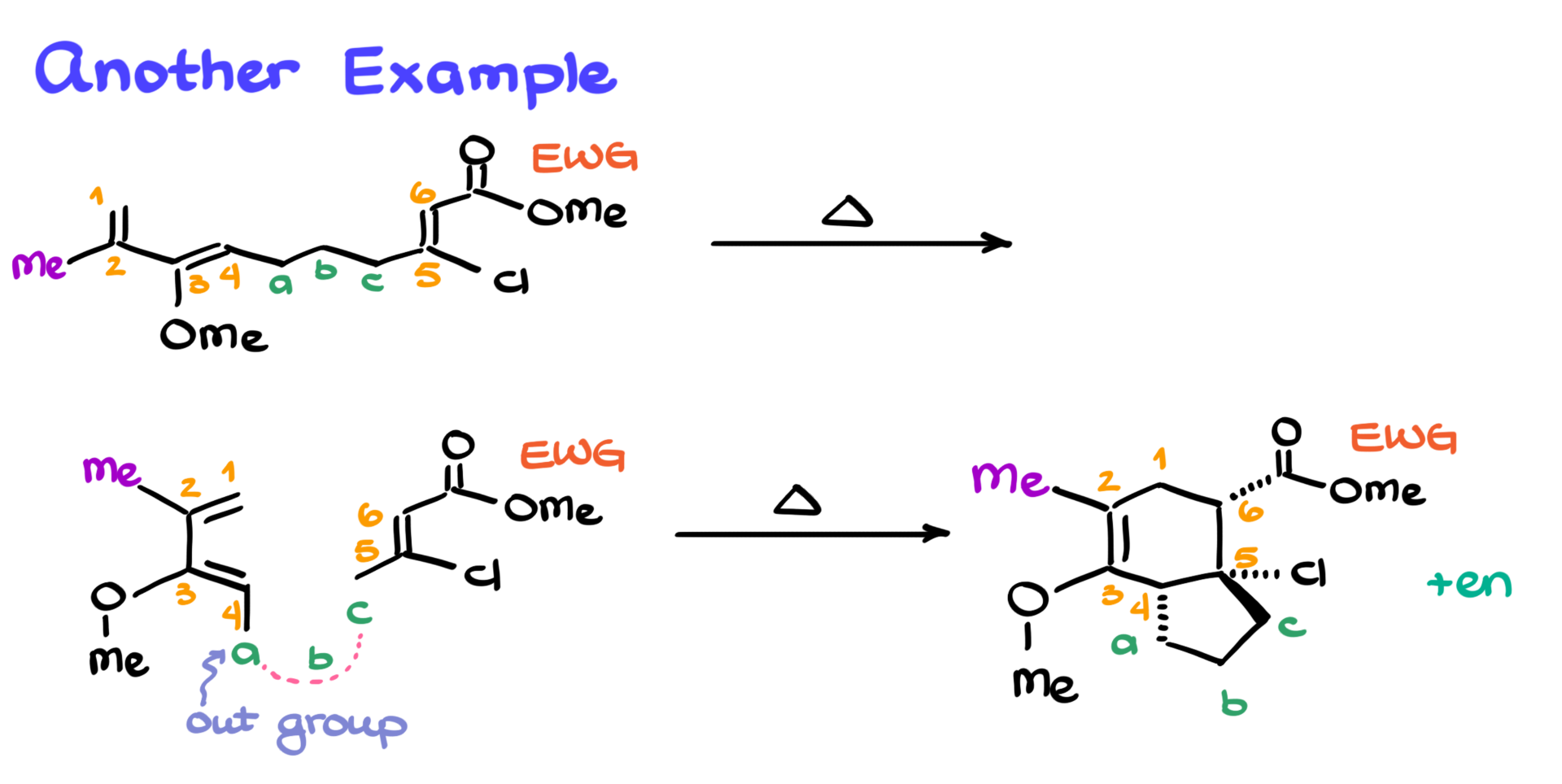
At first glance, it looks like a complete monstrosity, but I promise, if we follow the same steps as before, we’ll get the product just fine.
I’ve identified my diene on the left side of the molecule, so I’ll number it 1 through 4. Then I’ll number the dienophile as 5 and 6. The middle atoms—just three this time—I’ll call A, B, and C. No D this time. And, of course, I’ll note the electron-withdrawing group on atom 6.
Now I’m ready to start building the molecule. I’ll outline the diene in the s-cis conformation and start adding groups. On atom 2, there’s a methyl group, so I’ll show that. On atom 3, there’s a methoxy group, so I’ll draw that too.
Like before, I’ll place atom A, and from the original structure, I see it’s cis to the methoxy group, which means it’s the out-group again. So I’ll keep it cis in my sketch.
Next, I’ll move to the dienophile—atoms 5 and 6. On atom 5, there’s a chlorine, so I’ll draw that. Cis to the chlorine is the electron-withdrawing group, so I’ll add that too. Atom C is trans to the electron-withdrawing group, so I’ll show that on the opposite face. As before, I’ll mark where atom B goes without drawing it just yet.
Now, time to construct the final product. I’ll draw the six-membered ring with a double bond and number it 1 through 6, just like in any Diels-Alder reaction. On atoms 2 and 3, I’ll add the methyl and methoxy groups. Since these are sp²-hybridized carbons, there’s no stereochemistry to worry about—they can just go flat on the plane.
I’ll place the electron-withdrawing group on a dash again. Cis to that, I’ll put the chlorine on atom 5, also on a dash. Carbon C, which is trans to the electron-withdrawing group, will go on a wedge. Atom A, being cis, will go on a dash as well. I’ll finish by placing atom B and connecting the bonds.
This gives us the final product, plus the corresponding enantiomer from the reaction.
So, no matter how intimidating a molecule might look, as long as you follow these steps—this algorithm—you’ll be able to construct your final product without any problems and with the correct stereochemistry.
Now, the only thing left to do is practice!