Malonic Ester Synthetic Strategies
For any malonic ester synthesis, we start with a malonic ester—in this case, diethyl malonate. Through a series of enolate formation and alkyl substitution reactions, we introduce one or sometimes two alkyl groups. After that, we perform a decarboxylation reaction. This step eliminates one of the ester groups completely—let’s say the one on the right—while the other ester converts into a carboxylic acid.
This means that in the generic example I have on the screen, the final product is a carboxylic acid. The key takeaway is that the only parts of the original malonic ester that remain in the final product are the carbonyl group and the alpha-carbon adjacent to it.
Example 1
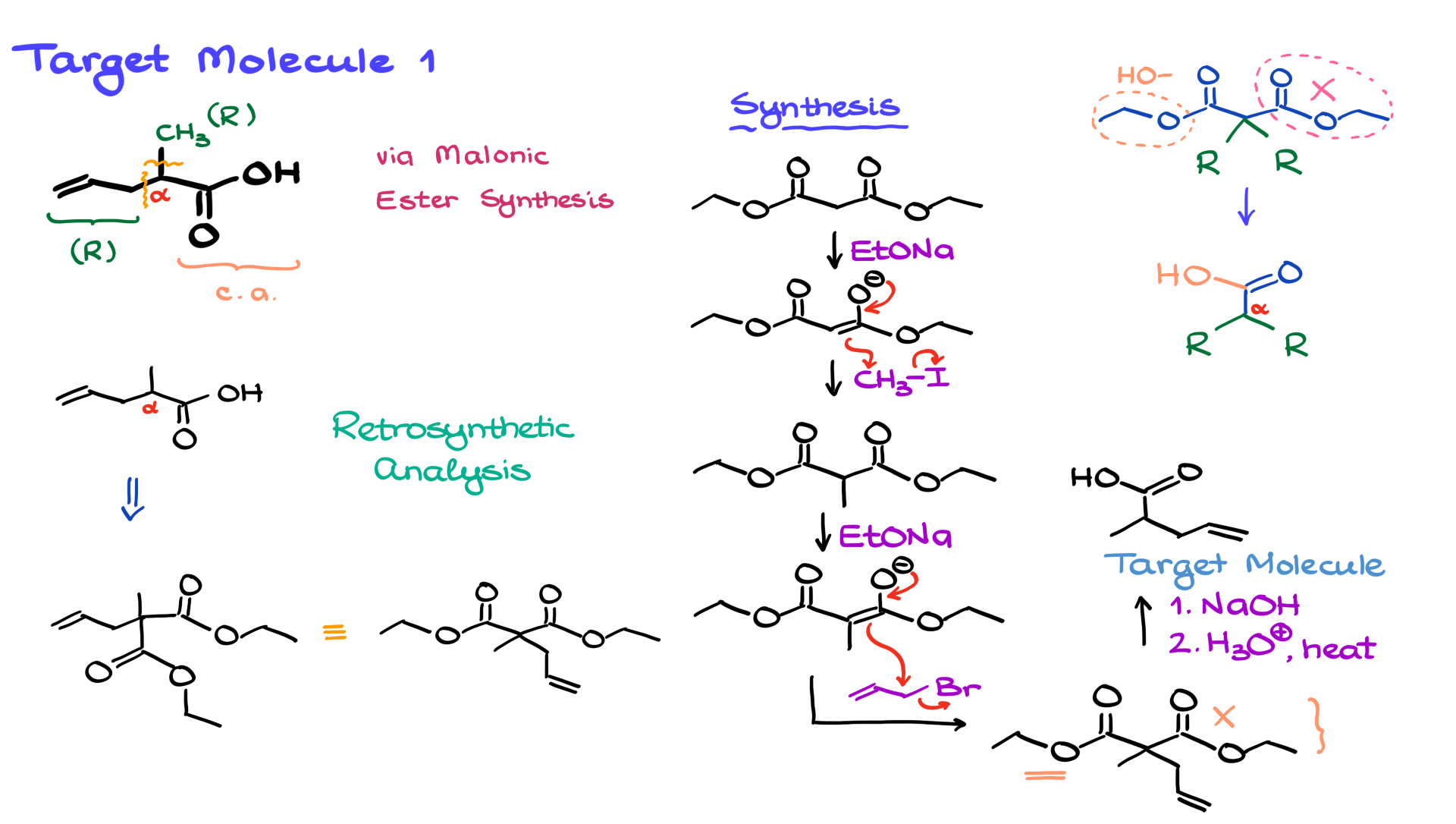
Now, looking at our target molecule, we can identify the carboxylic acid functional group right here. That part originates from the malonic ester. Next, we have the alpha-carbon adjacent to the carbonyl, which also comes from the malonic ester. However, two new carbon-carbon bonds have formed at the alpha position. One of them is connected to a methyl group (CH₃), which is one of our R groups, while the other is an allyl group.
Now, using the retrosynthetic approach we just outlined, let’s redraw our target molecule. The first thing to note is that the alpha position originally had a second ester group attached to it. This means that the immediate precursor to our molecule was an ester, which looked something like this. One ester group—let’s say this one—eventually becomes the carboxylic acid, while the other ester is lost during decarboxylation.
The ethyl groups on the esters are irrelevant. They could be ethyl, methyl, propyl—whatever. I used ethyl here because it’s common. Now, if we redraw the structure in a more familiar form, it clearly resembles the malonic ester structure we expect, showing our two alkyl groups: the methyl and the allyl group.
With the retrosynthetic analysis complete, let’s move on to the actual synthesis.
We begin with diethyl malonate and deprotonate it with a base that matches the alkyl group of the ester, forming the corresponding enolate. Next, we introduce the first alkyl group. It doesn’t really matter which one we add first, but let’s start with methyl iodide. The enolate we just formed undergoes an SN2 reaction with methyl iodide, giving the first alkylated product.
Now, we repeat the enolate formation step, generating another nucleophile. This time, we introduce the second electrophile—our allyl bromide (though the halide identity isn’t critical). Another SN2 reaction occurs, incorporating the allyl group.
At this stage, we’re just one step away from our target molecule. We now need to perform hydrolysis and decarboxylation. As I mentioned in my video on malonic ester synthesis, there are two ways to accomplish this. Here, I’ll use the mixed method—first performing saponification under basic conditions, followed by neutralization and acidic decarboxylation. This eliminates one ester and converts the other into the corresponding carboxylic acid, yielding our target molecule. Pretty straightforward!
Example 2
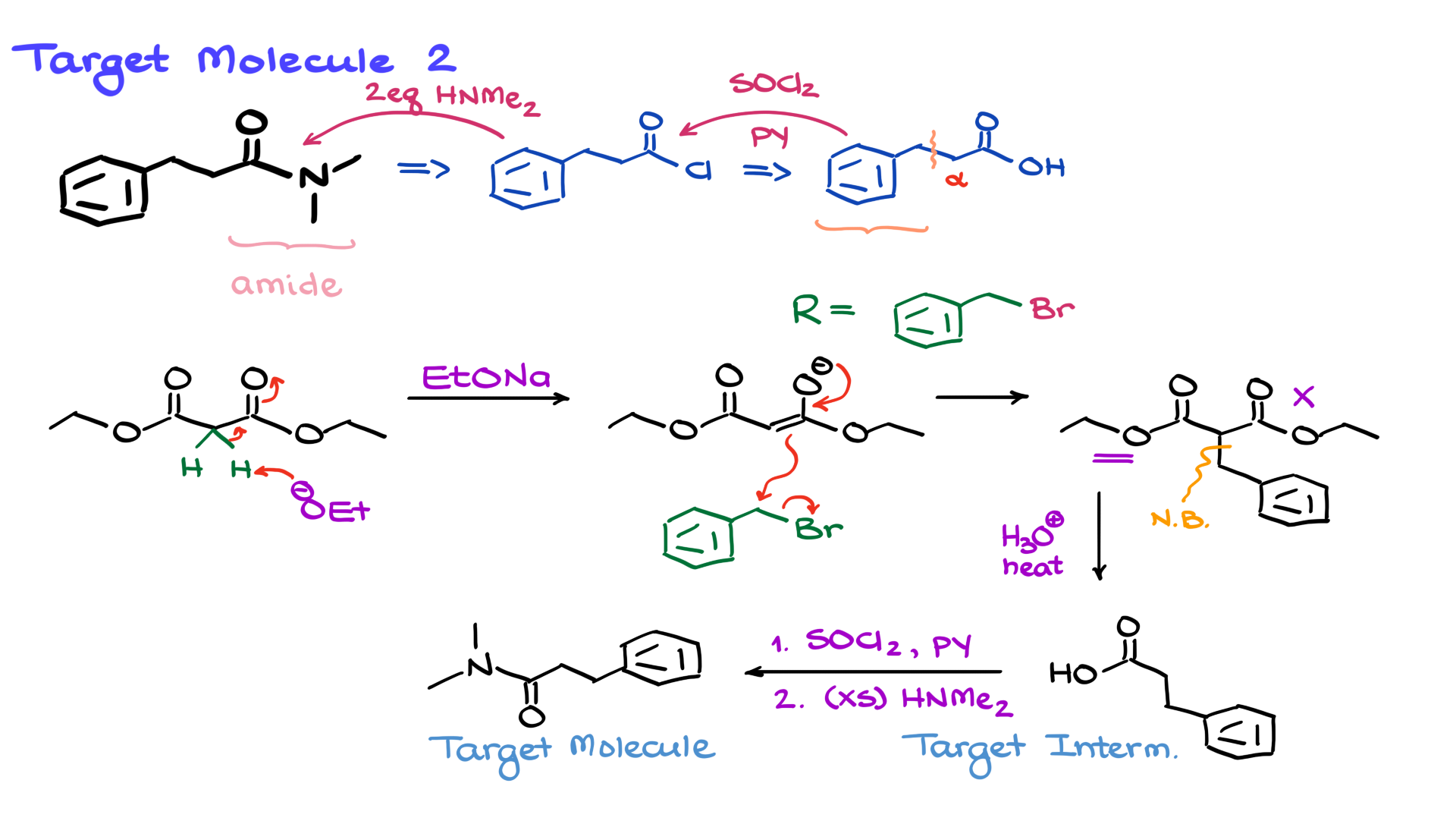
Now, let’s look at another example. In this case, our target molecule doesn’t contain a carboxylic acid; instead, it has an amide functional group. But that’s not a problem! We can easily convert carboxylic acids into amides—or more precisely, we convert carboxylic acids into acid chlorides, which then react to form amides.
So, the immediate precursor to this amide was the corresponding acid chloride, which, in turn, came from a carboxylic acid. The transformation from carboxylic acid to acid chloride is typically done using thionyl chloride (SOCl₂) in pyridine. Then, to convert the acid chloride into an amide, we use two equivalents of dimethylamine.
Now that we’ve identified the carboxylic acid precursor, our question shifts: how do we synthesize this carboxylic acid using malonic ester synthesis?
Just like before, we focus on the alpha-carbon. Here, we see that our R group is a benzyl group. That tells us that our alkyl halide precursor must have been a benzyl bromide (or another similar halide). With that information, we can complete our synthesis right away.
We start with diethyl malonate and deprotonate it using a base, forming the enolate. Then, we introduce our benzyl halide as the electrophile, which undergoes an SN2 reaction with the enolate. This forms our alkylated product, with a new carbon-carbon bond at the alpha position.
Since we only need to add one R group, the final step is decarboxylation. To keep things varied, this time, I’ll use a purely acidic decarboxylation. First, we hydrolyze the esters to carboxylic acids, and then one carboxyl group is eliminated. The remaining carboxyl group forms our desired carboxylic acid.
From here, we already know how to convert the carboxylic acid into an amide: first, react it with SOCl₂ in pyridine to form the acid chloride, and then treat it with excess dimethylamine. This gives us our final target molecule.
So, just because a molecule doesn’t initially appear to be a carboxylic acid doesn’t mean we can’t use malonic ester synthesis. If we understand functional group transformations, we can use malonic ester synthesis to create a variety of functional groups that can be derived from carboxylic acids.
Example 3
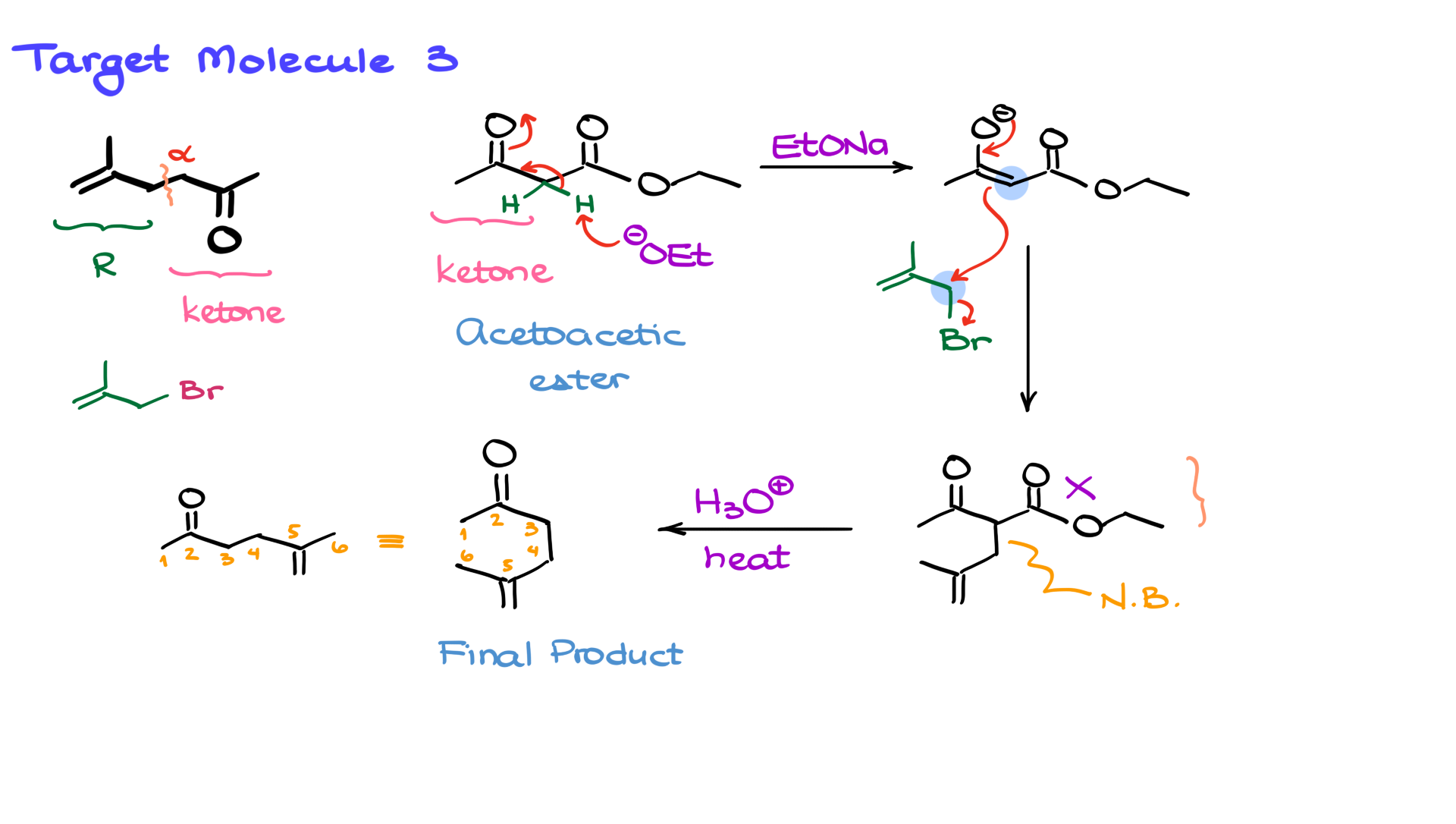
Finally, let’s look at one more example. This time, our target molecule is not a carboxylic acid or an amide—it’s a ketone. Can we still use malonic ester synthesis? Actually, yes! But instead of malonic ester synthesis, we’ll use acetoacetic ester synthesis, which is a closely related method.
The key difference is that instead of starting with a malonic ester, we use a β-keto ester—specifically, ethyl acetoacetate. This approach is ideal when synthesizing ketones.
The strategy remains the same. We examine the alpha position and identify the R group attached to it. In this case, the R group is on the left side. That means our alkyl halide precursor must have had this structure—let’s assume it was a bromide, as before.
The synthetic approach mirrors the previous cases. We begin with enolate formation using sodium ethoxide, generating a nucleophilic enolate. Next, we introduce the alkyl halide, which undergoes SN2 substitution, forming a new carbon-carbon bond.
Finally, we perform decarboxylation. Just like before, I’ll use acidic conditions to remove the ester group, yielding our final ketone product. We can also represent the molecule in a more linear form, but the connectivity remains the same.
As you can see, malonic ester synthesis—and its counterpart, acetoacetic ester synthesis—are incredibly versatile techniques. They allow us to construct a wide variety of molecules, including carboxylic acids, amides, and ketones, simply by modifying the functional groups through subsequent transformations.