Intermolecular Forces in Organic Chemistry
In this tutorial, I want to talk about the intermolecular forces and how the 3D shape of a molecule affects them.
So, what are the intermolecular forces? The intermolecular forces are the weak forces that occur between the molecules. They are not as strong as chemical bonds. And they are not as permanent either and can shift easily.
London Dispersion
The first intermolecular force I want to talk about is the London dispersion force. This type of interaction occurs when two molecules approach each other and the electron clouds on the atoms experience a slight polarization. Because of that, the London dispersion forces are sometimes called induced dipole interactions.
While the dispersion forces are weak, they do scale with the molecule size and atomic weights. So, bigger molecules can have a very large dispersion interaction merely due to their size or weight.
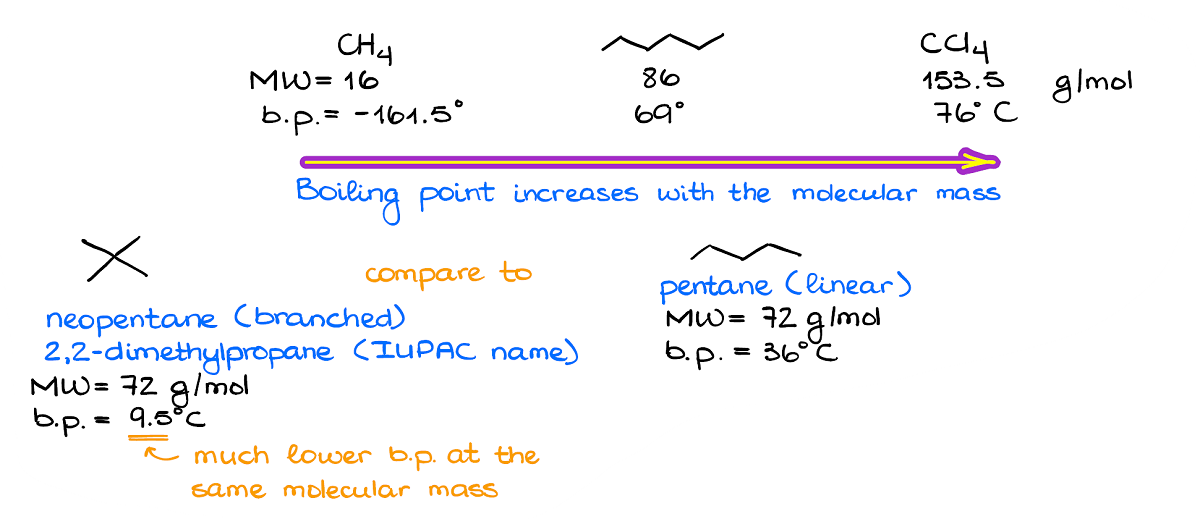
Above, there’s an example where I have three molecules with different molecular masses. These molecules experience no other intermolecular forces but the London dispersion. And looking at the boiling points of the molecules, we can see that there’s a relationship between the molecular mass and the boiling point.
There’s one other aspect of the dispersion forces we need to keep in mind. The linear molecules are generally more polarizable than the branched ones with the same molecular mass. So, if we compare the neopentane and regular pentane, we’ll see that although they both have exactly the same molecular mass the boiling points are quite different. And the branched isomer is a gas at room temperature, while the linear isomer is a liquid.
Normally, we’ll only consider how linear or how branched the molecule is when everything else is the same or almost the same. For instance, if you have a drastic difference between the molecular weights, then the fact that one molecule has more branches than the other one will have a negligible effect.
Dipole-Dipole Interactions
The next important intermolecular force is the dipole-dipole interaction. Unlike the London dispersion, we see the dipole-dipole interactions when we have permanent dipoles in the molecule.
So, what are the permanent dipoles?
We have permanent dipoles when we have polar bonds and those bonds are oriented in space in such a manner as not to cancel each other out. And since dipoles put a partial positive charge and a partial negative charge on the opposite ends on the molecule (or a part of a molecule), they experience electrostatic attraction.
Let me illustrate it with an example.
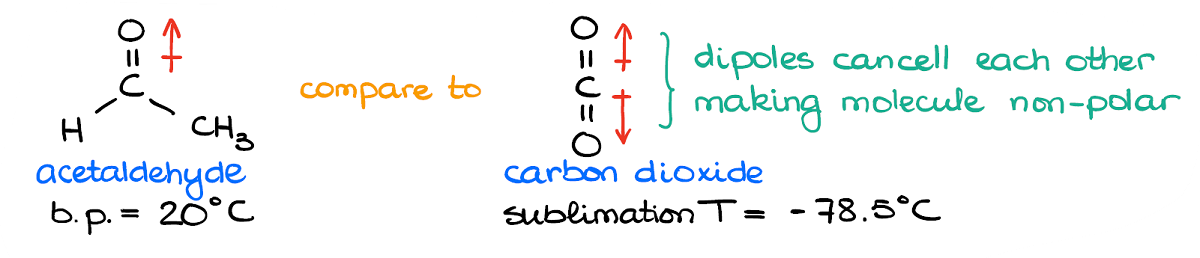
Here I have a molecule of an acetaldehyde and a molecule of carbon dioxide. In the acetaldehyde example I have the C=O bond with is a permanent dipole. We have a significant partial negative charge on the oxygen atom, and a sizeable positive charge on the carbon atom. Nothing needs to polarize this molecule—it’s already a dipole.
In comparison, the carbon dioxide molecule has two C=O bonds. But since the molecule has the linear geometry, those bonds are pointing in the opposite directions. And while each bond puts a significant partial negative charge on the oxygens and a partial positive charge on carbon, they cancel each other making the molecule overall non-polar.
Hydrogen Bonding
Finally, we get to the hydrogen bonding.
The hydrogen bonding is a special type of dipole interaction. It’s generally much stronger than most dipole interactions and it has a more complex nature than a simple electrostatic attraction. Hydrogen bonding occurs when a hydrogen connected to an N, O, or F atoms interacts with an electron pair from another N, O, or F atom.
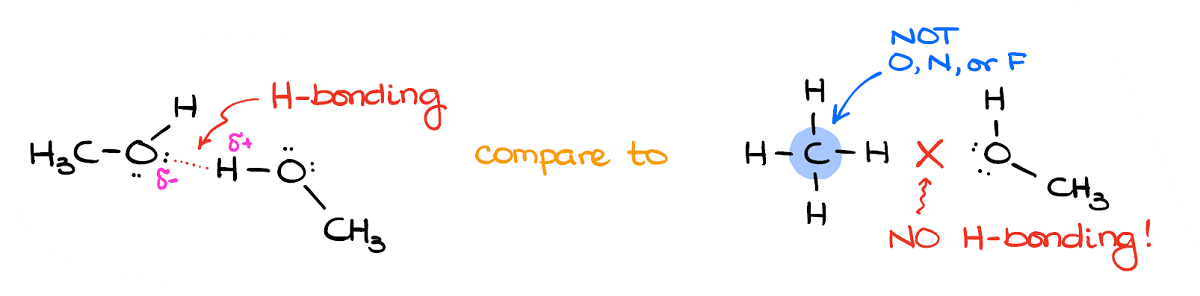
Here, in the first example we have two -OH groups interacting with each other. Notice, how one of the H’s is attracted to the oxygen atom of another molecule. Hydrogen bonding is roughly 1/10th of a typical covalent bond in strength. So, having enough of those in a molecule can make the molecules stick to each other pretty tightly. For instance, the DNA, RNA, and protein molecules are held together predominantly by the hydrogen bonding.
Always remember, that the H atom must be attached to N, O, or F for the hydrogen bonding to occur. So, if your hydrogen is connected to another atom, like for instance we have H attached to C in the right example, there will be no hydrogen bonding between those two molecules.
Here’s a shortcut for you. If you’re looking for hydrogen bonding, always look of the -OH or NH groups. Those can serve as both hydrogen bond donors and hydrogen bond acceptors.
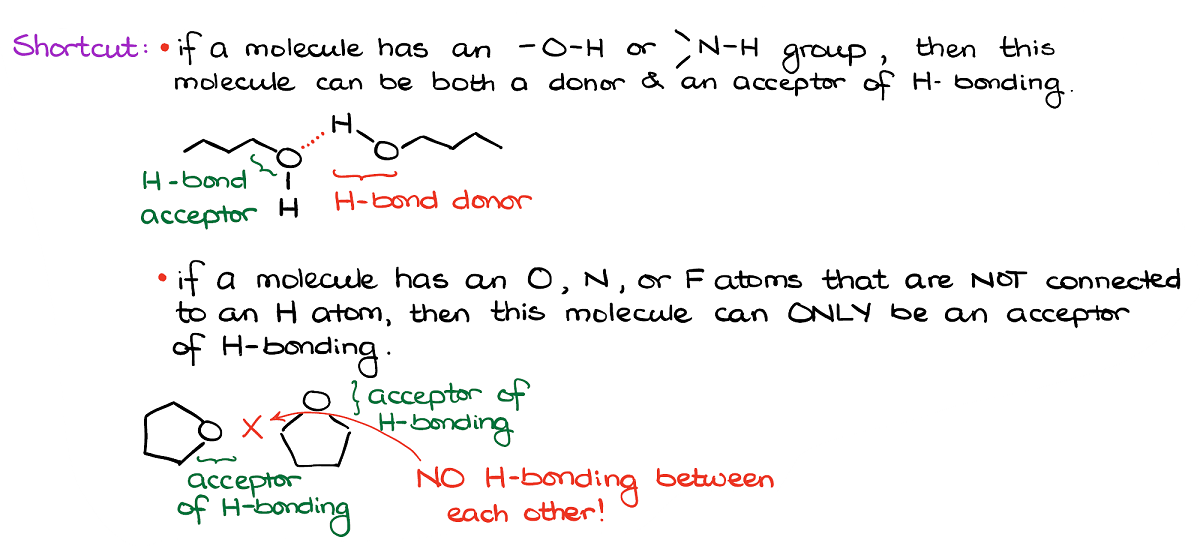
If, however, your molecule has O’s or N’s but they don’t have hydrogens connected to them, those can only be the acceptors of hydrogen bonding.
Example of the Typical Exam Question
When it comes to the exam questions, you’re typically going to have to rank the molecules according to their boiling points by looking at the molecular structure.
So, here’s an example of a lineup like that.

The very first thing you want to check is the molecular masses for your compounds.
Generally, instructors will give you molecules with similar masses, so that they all have the same amount of the dispersion interactions. However, I’ve seen a few exams where instructors threw a mean curved ball at students giving them a completely non-polar yet a massive molecule which ended up with the highest boiling point. So, always double check just in case.
As I’ve mentioned a moment ago, all molecules will always have London dispersion. So, for as long as your molecular weights are close (say, within 10% or so), the differences in the dispersion forces will be negligible.
Next, by checking for the polar bonds and the dipole vectors, we can see that we have a clearly non-polar molecule: cyclopentane.
Finally, we want to check for the hydrogen bonding. While THF and butanal can be acceptors of the hydrogen bonding, they cannot be the donors of it. So, we won’t have any H-bonding in a pure sample of either of those. Thus, we have a clear leader in terms of the most intermolecular interactions. So, according to our analysis here, the molecule on the right—butanol—should have the highest boiling point.
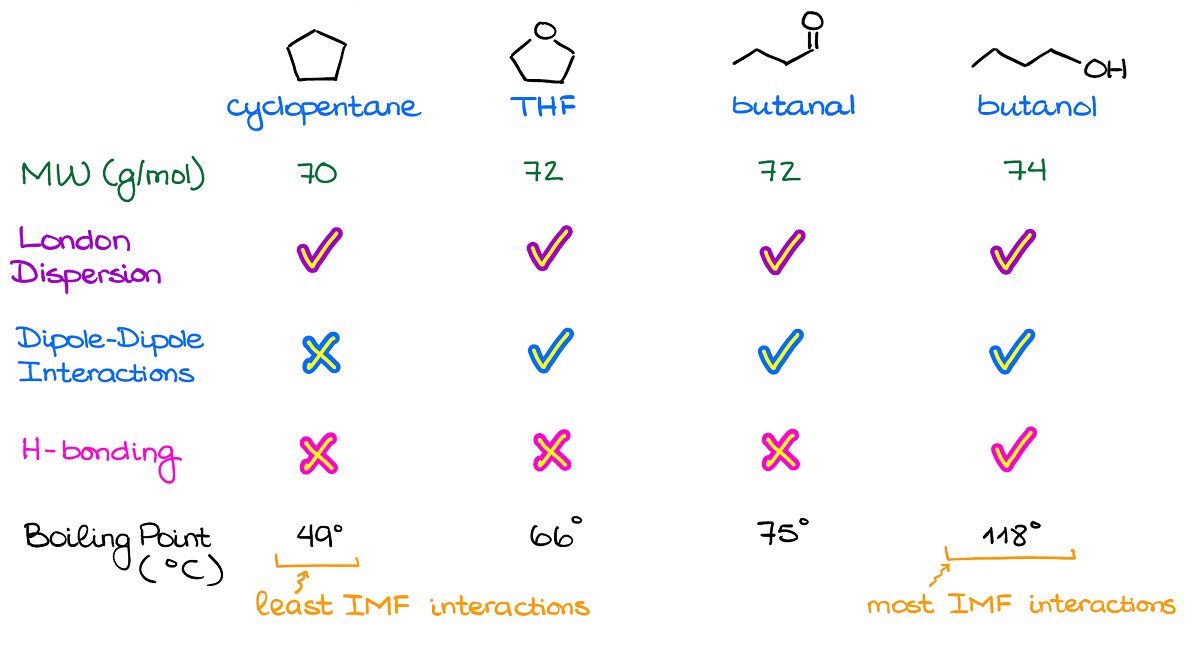
And by checking the values, we see that it is indeed correct.
Now, you may be wondering why THF has a lower boiling point than butanal while they have the same intermolecular forces and exactly the same molecular mass. This is where the molecular structure comes into play again. In THF, we have oxygen in the bent molecular geometry, thus the dipoles will be at an angle to each other diminishing the overall dipole. In the butanal, however, the molecular geometry around oxygen is linear, so the dipole vector is going to be rather large making it more polar than THF.
Like with any type of material in organic chemistry, practice makes it perfect. So, make sure you work through a few of those ranking problems to get the skill ready for the next test or exam.