Hofmann and Curtius Rearrangements
In this tutorial, I want to talk about Hofmann and Curtius rearrangements, which are very old yet useful methods for converting carboxylic acids into primary amines while cutting one carbon off from the chain in the process.
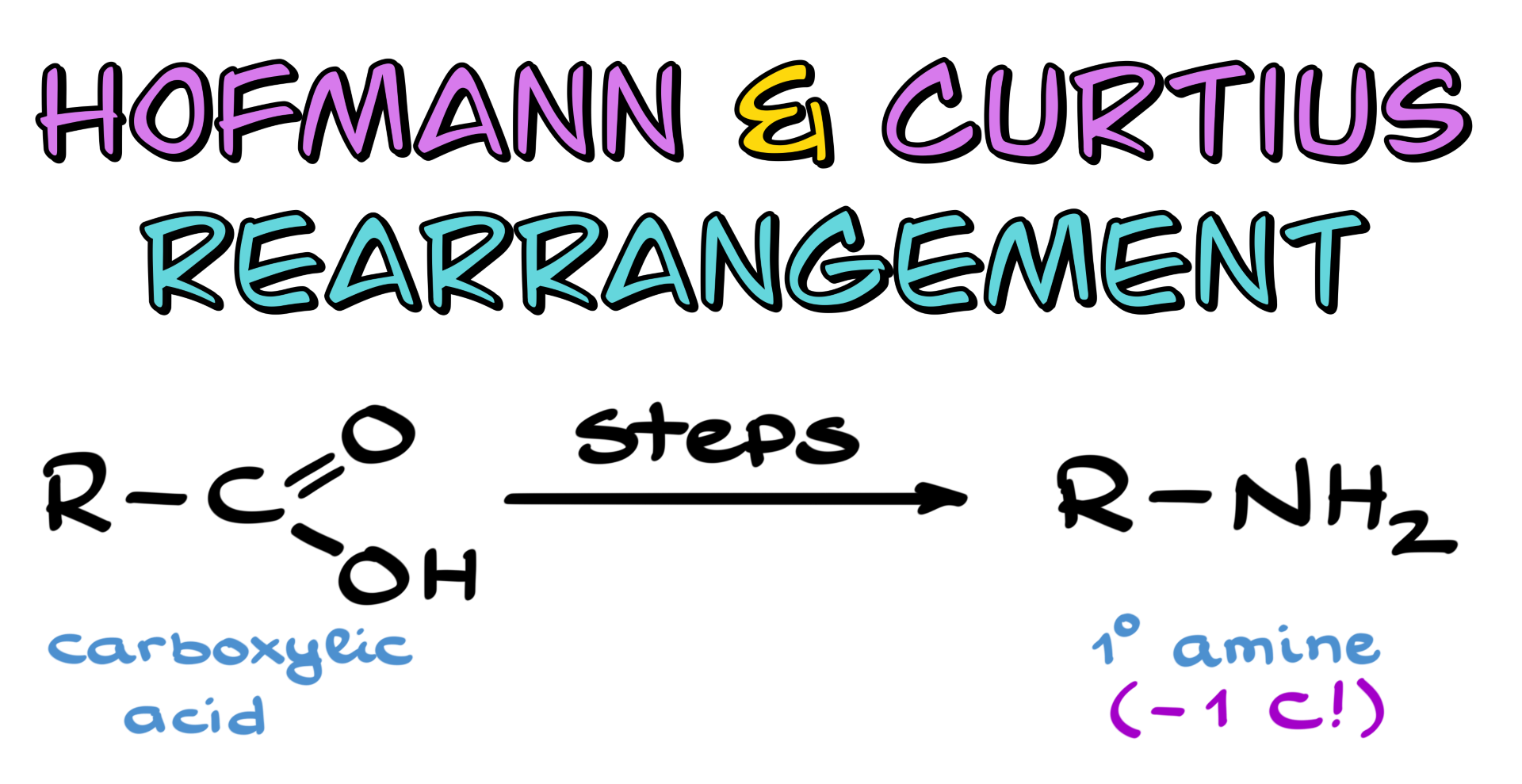
General Scheme of Hofmann and Curtius Rearrangements
The way this scheme works is that we start with a carboxylic acid, convert it into the acid chloride, and from that point, we reach a fork in the road. If we convert the carboxylic acid into the corresponding amide, we follow the Hofmann pathway. However, if we convert the acid chloride into the corresponding azide, that leads us down the Curtius pathway.
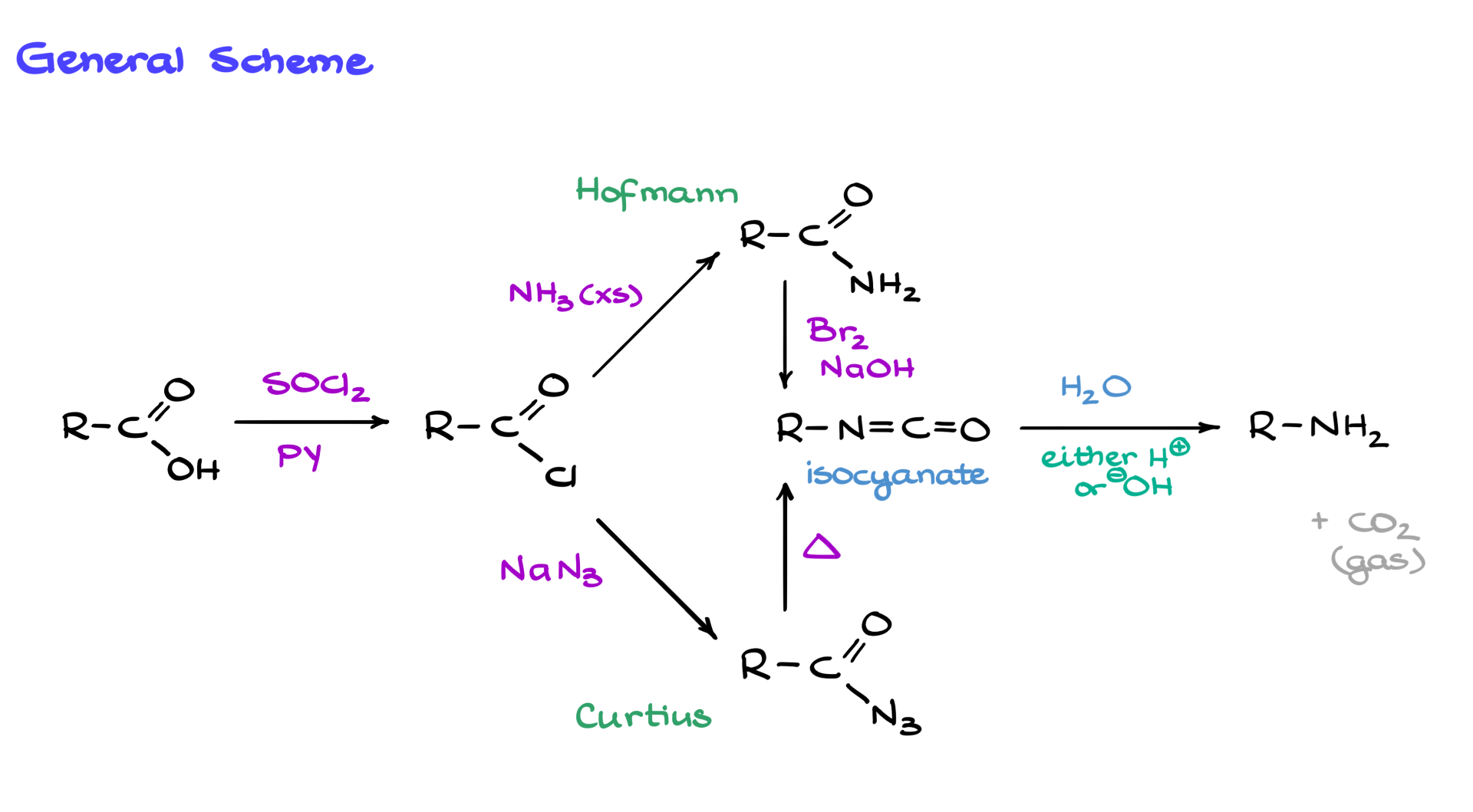
Regardless of which pathway we choose, we ultimately form an isocyanate, which, after hydrolysis—either acidic or basic—yields the corresponding amine and carbon dioxide. The CO₂ typically just escapes as a gas unless we are working under basic conditions, in which case it forms a carbonate, but I digress. Since this scheme involves several moving parts, let’s look at each reaction individually.
Formation of Acid Chloride
To begin, let’s examine the formation of the acid chloride.
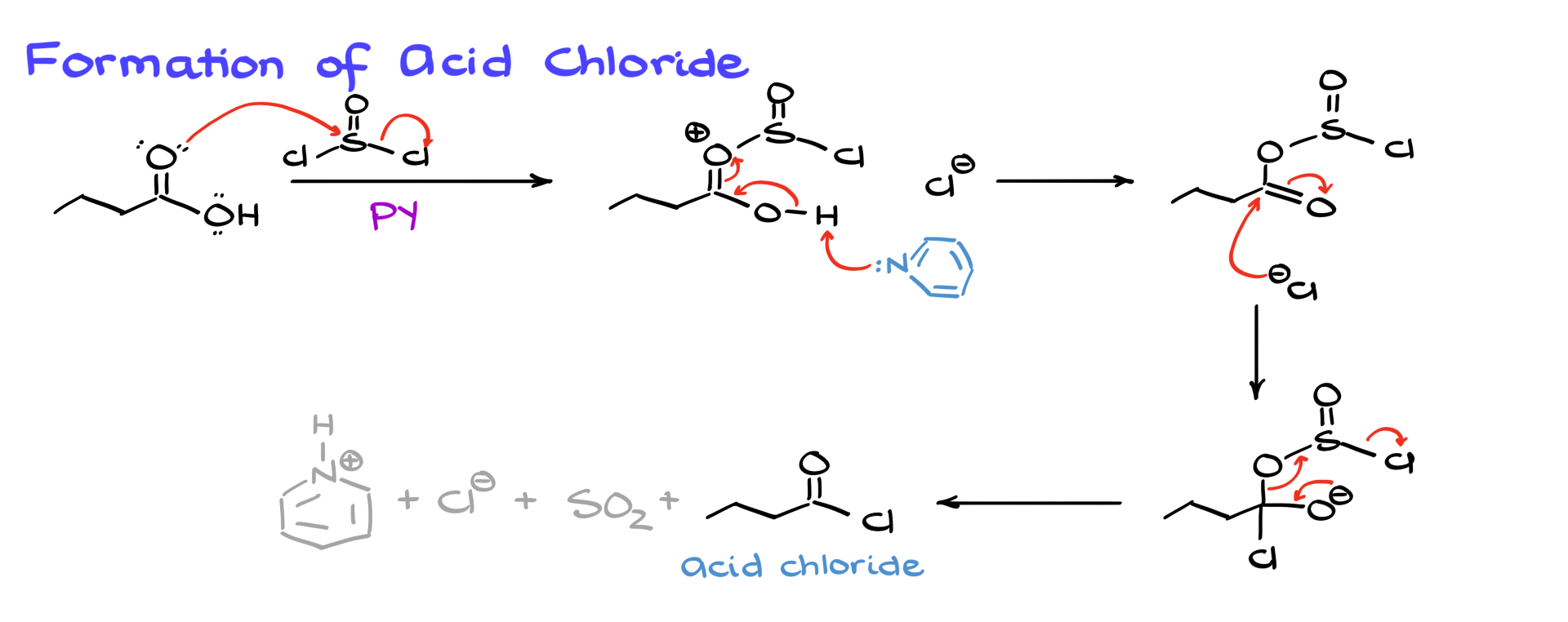
While there are many ways to synthesize acid chlorides, the method using thionyl chloride (SOCl₂) is probably the most common. The reaction begins with a nucleophilic attack by the carboxylic acid on thionyl chloride, kicking out one of the chlorines and forming an intermediate along with Cl⁻. From this point, the reaction can proceed through a couple of different pathways. I’ll show the one where pyridine comes in and deprotonates the intermediate, yielding a derivative that is then attacked by Cl⁻ at the carbonyl, forming a tetrahedral intermediate. This intermediate then collapses, kicking out the sulfur-containing moiety and leaving us with the acid chloride, SO₂, CO₂, and pyridinium chloride as byproducts.
There’s an alternative way to depict this mechanism, where Cl⁻ attacks first, followed by deprotonation, but in the end, both approaches yield the same acid chloride. If your instructor prefers one over the other, just go with what they like—after all, I’m not the one grading your exams.
Hofmann Rearrangement
Now that we have our acid chloride, let’s move on to the Hofmann pathway.
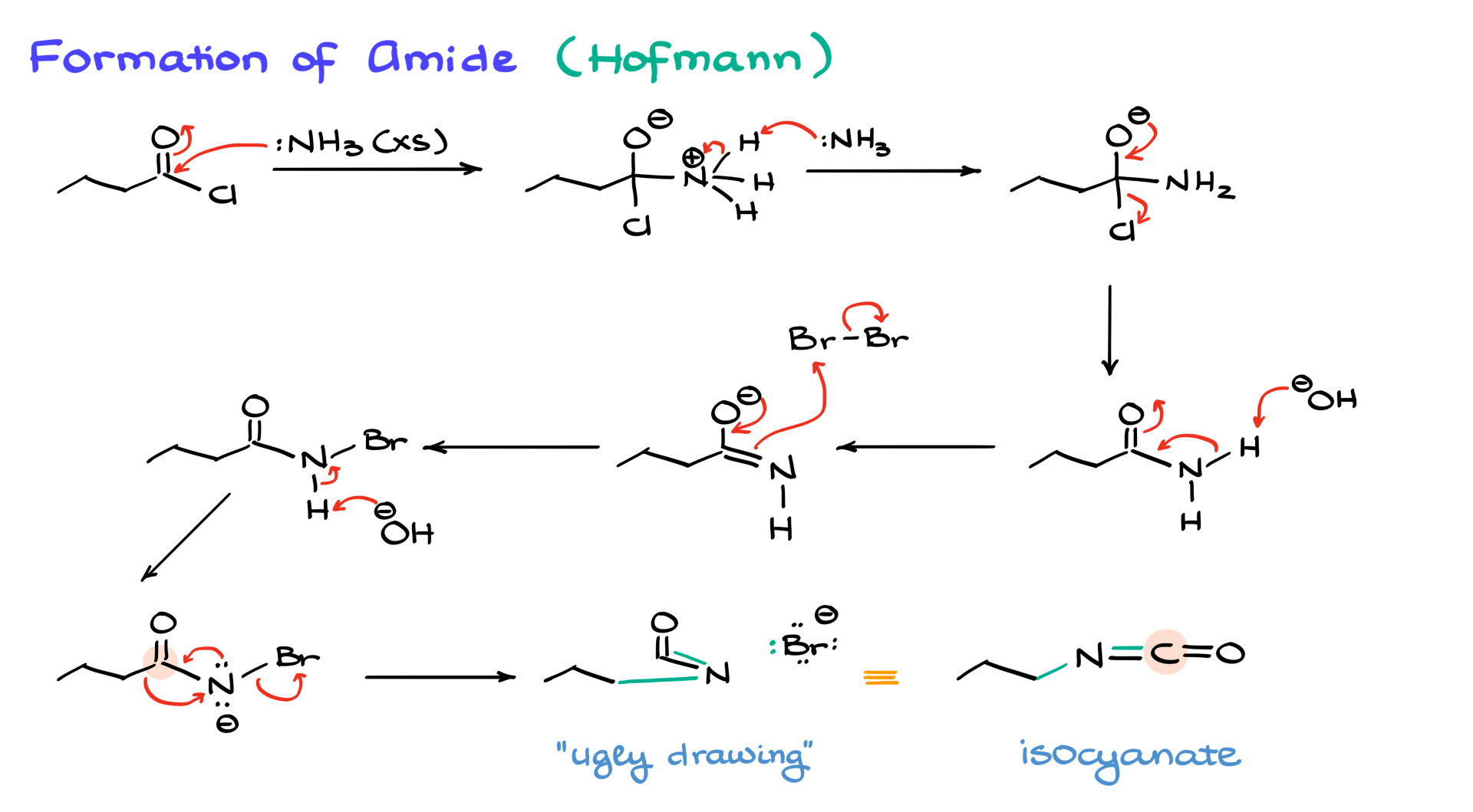
The first step here is the formation of the amide, which is just a typical acyl substitution reaction. Ammonia attacks the carbonyl, forming a tetrahedral intermediate. A second equivalent of ammonia, which acts as a base, then facilitates the departure of chlorine, yielding the amide.
Returning to the general scheme, once we form the amide in the Hofmann pathway, the next step involves reacting it with Br₂ in the presence of sodium hydroxide. Hydroxide deprotonates the amide, forming a negatively charged intermediate. This intermediate is a good nucleophile, and since we have an electrophile (Br₂) present, they react, forming an N-bromoamide. This step is reminiscent of enolate halogenation under basic conditions.
At this point, the nitrogen’s remaining proton is even more acidic due to the electron-withdrawing effect of bromine. The second equivalent of base then deprotonates nitrogen, forming an unstable intermediate that immediately undergoes rearrangement. In this rearrangement, the alkyl group migrates to nitrogen, bromine leaves, and nitrogen forms a double bond with carbon, resulting in the isocyanate intermediate. If you look at the structure, you’ll notice that the original four-carbon chain has now lost one carbon, which will be eliminated in the hydrolysis step.
Curtius Rearrangement
Now, let’s examine how the reaction proceeds via the Curtius pathway.
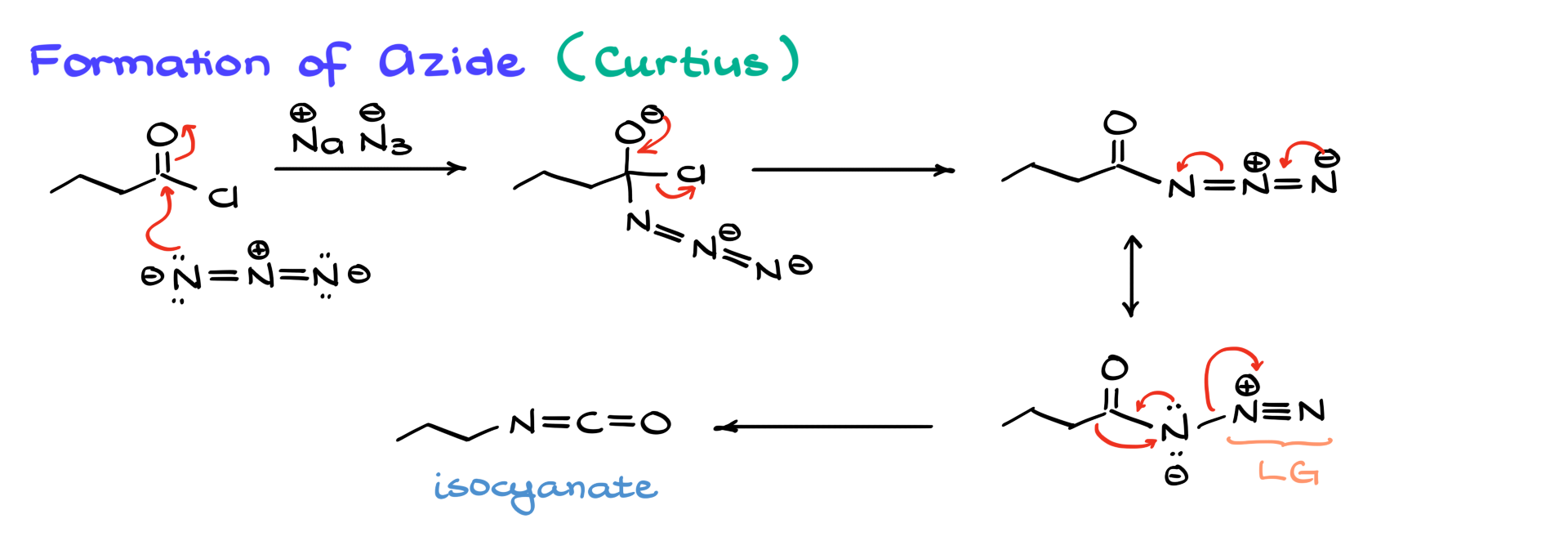
In this pathway, azide (N₃⁻) is an excellent nucleophile, so it directly attacks the carbonyl of the acid chloride, forming a tetrahedral intermediate. This intermediate then collapses, kicking out chlorine and forming the organic azide. From this point, the reaction simply requires heating—no additional reagents.
To better understand what happens next, let’s consider an alternative resonance structure of the azide. If we visualize the electrons moving around, we get a species that looks remarkably similar to the N-bromoamide from the Hofmann rearrangement. The key takeaway here is that, just like Br⁻, N₂ is an excellent leaving group. This means that we observe the same rearrangement mechanism as before—alkyl migration to nitrogen, loss of N₂ as a gas, and formation of the isocyanate intermediate. So regardless of whether we follow the Hofmann or Curtius pathway, we always end up with the same isocyanate, which then undergoes hydrolysis.
Isocyanate Hydrolysis
Hydrolysis can occur under either acidic or basic conditions, though slightly acidic conditions are often preferred.
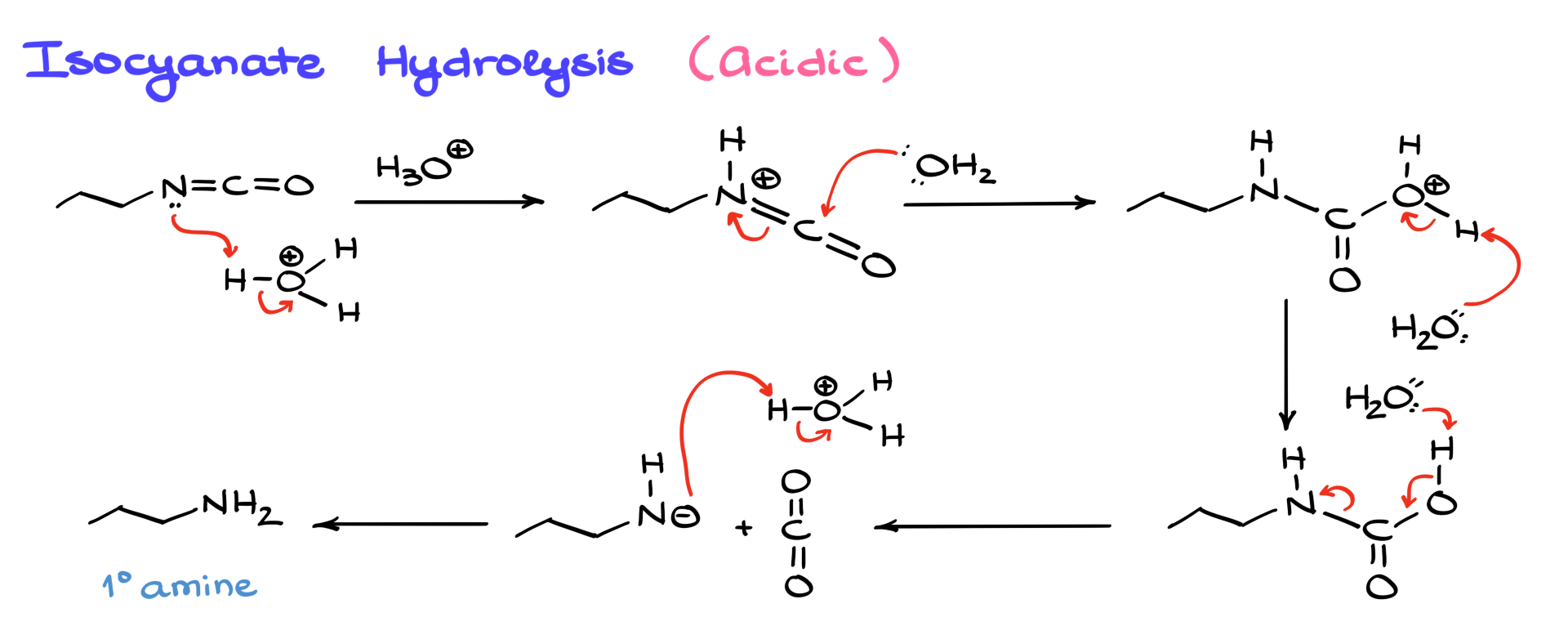
In acidic hydrolysis, the first step is protonation of the isocyanate, making it more electrophilic. Water then attacks, forming a protonated carbamic acid intermediate. A subsequent proton transfer leads to CO₂ release and the formation of the primary amine. Some may question the presence of a transient negatively charged nitrogen species, but because CO₂ rapidly escapes as a gas, this step is irreversible and entropy-driven, making it reasonable despite appearing unusual.
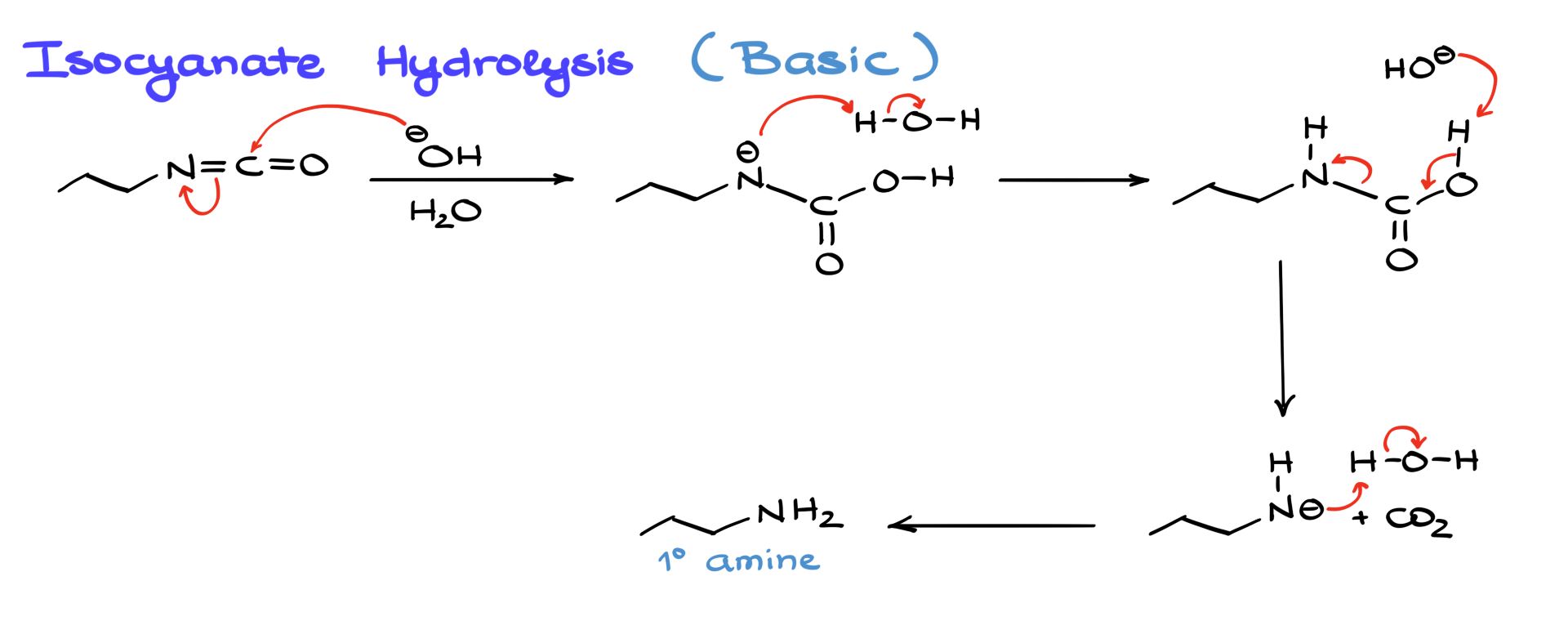
Alternatively, in basic hydrolysis, hydroxide attacks the isocyanate, forming a negatively charged nitrogen intermediate. This intermediate is then protonated by water, regenerating the carbamic acid, which subsequently decomposes into CO₂ and the primary amine. The end result is the same, but acidic hydrolysis is the more commonly used method.
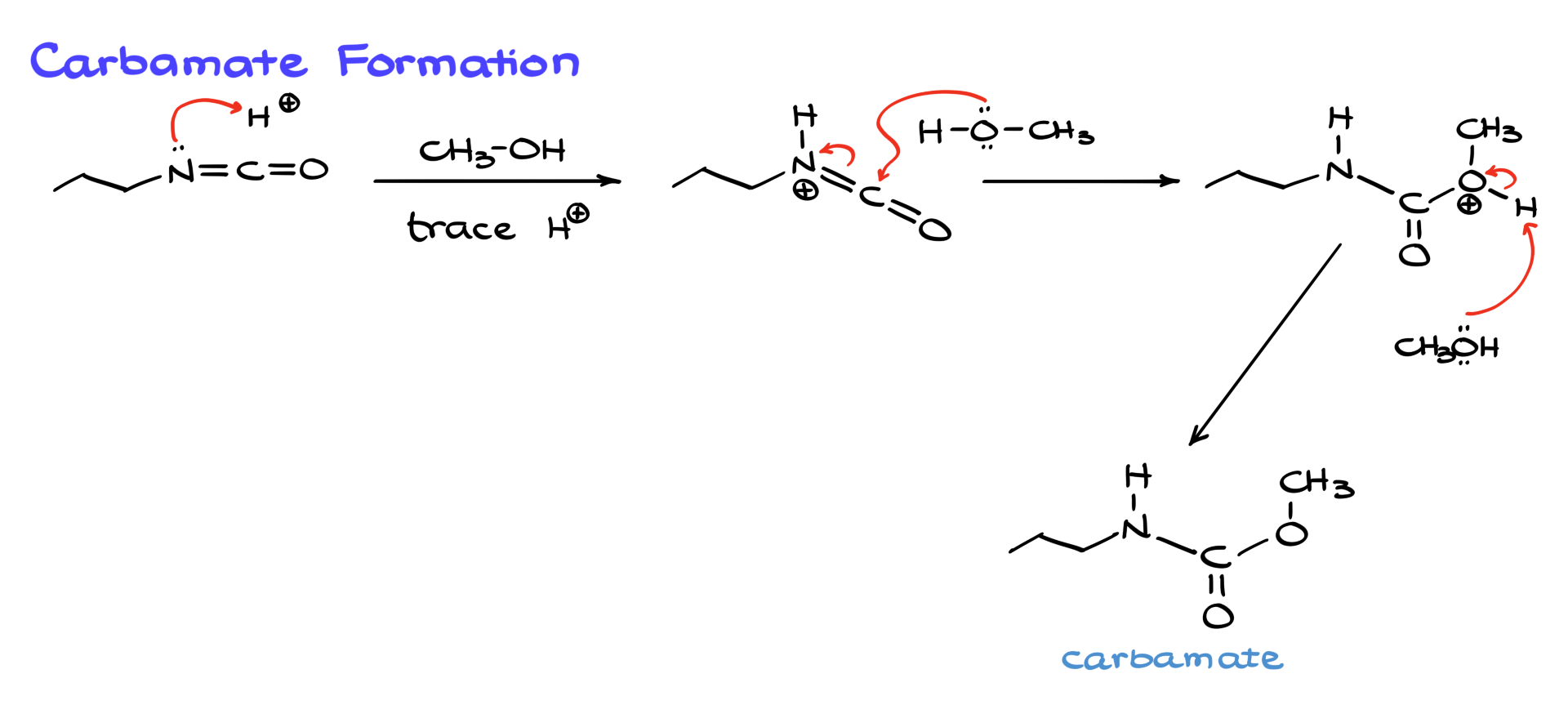
In addition to aqueous hydrolysis, isocyanates can also be treated with alcohols to form carbamates. This reaction, typically carried out with a trace of acid or base, follows a similar mechanism. The isocyanate is first protonated, making it more electrophilic, and then alcohol attacks the carbonyl, forming a protonated intermediate. A second equivalent of alcohol removes a proton, yielding the final carbamate product, which is a somewhat useful functional group in organic chemistry.
Recap
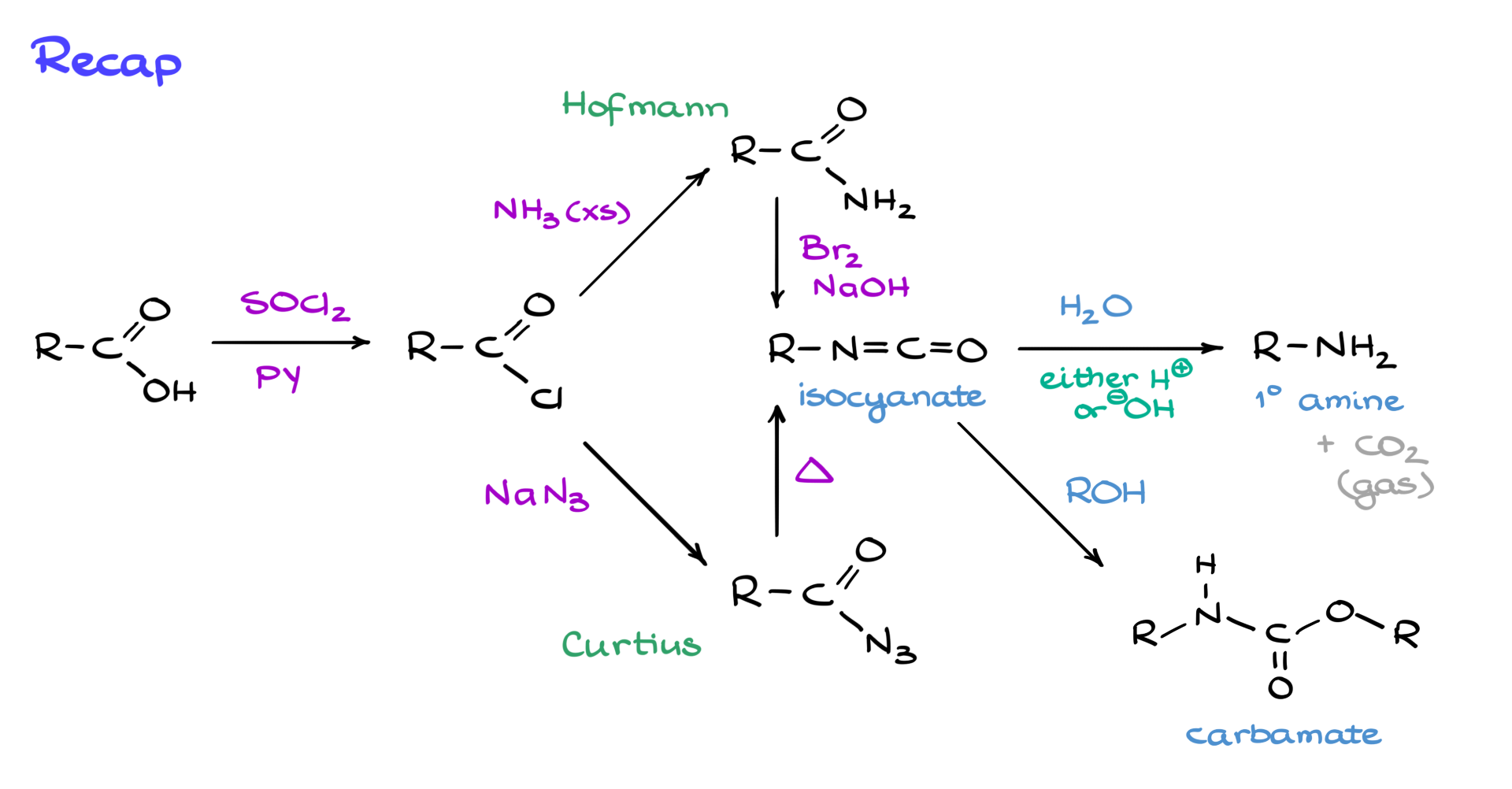
Looking back at our overall reaction scheme, it’s clear that Hofmann and Curtius rearrangements are nearly identical in mechanism. They provide a neat way to convert carboxylic acids into the corresponding amines while losing one carbon in the process. These rearrangements work for nearly any carboxylic acid, but they can be temperamental, sometimes leading to side products and poor yields. Nevertheless, for introductory organic chemistry courses, they remain a viable choice for multistep synthesis. Just remember to account for the loss of one carbon in your retrosynthetic planning.