Dieckmann Condensation
In this tutorial, I want to talk about the Dieckmann condensation, which is essentially the same as the Claisen condensation but in an intramolecular version that gives you a cyclic product at the end.
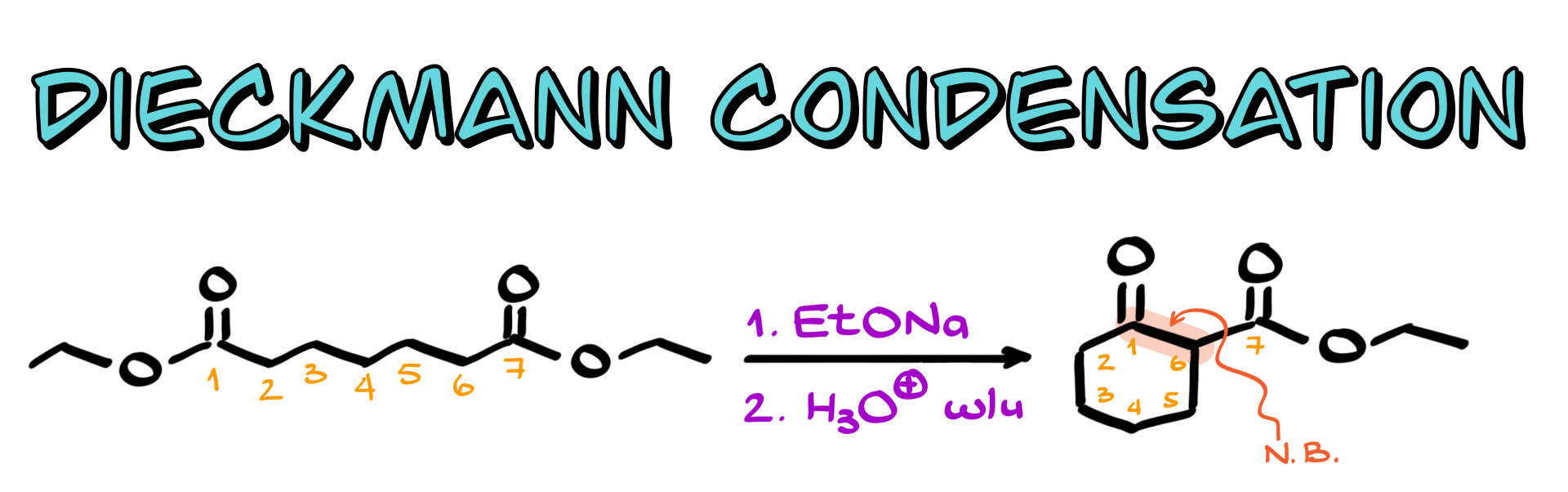
Unlike the Claisen condensation, where we start with two equivalents of esters—whether they are the same molecule or different—in the Dieckmann condensation, both ester groups are part of the same molecule. As a result, you end up with a cyclic compound, and while you still form a 1,3-dicarbonyl, the carbonyls are part of the cyclic moiety rather than sitting on a straight chain.
Mechanism of the Dieckmann Condensation
Let’s dive into the mechanism of this reaction. Just like in the Claisen condensation, we start by treating our molecule with a base—in this case, sodium ethoxide—which deprotonates one of the alpha positions of the molecule.
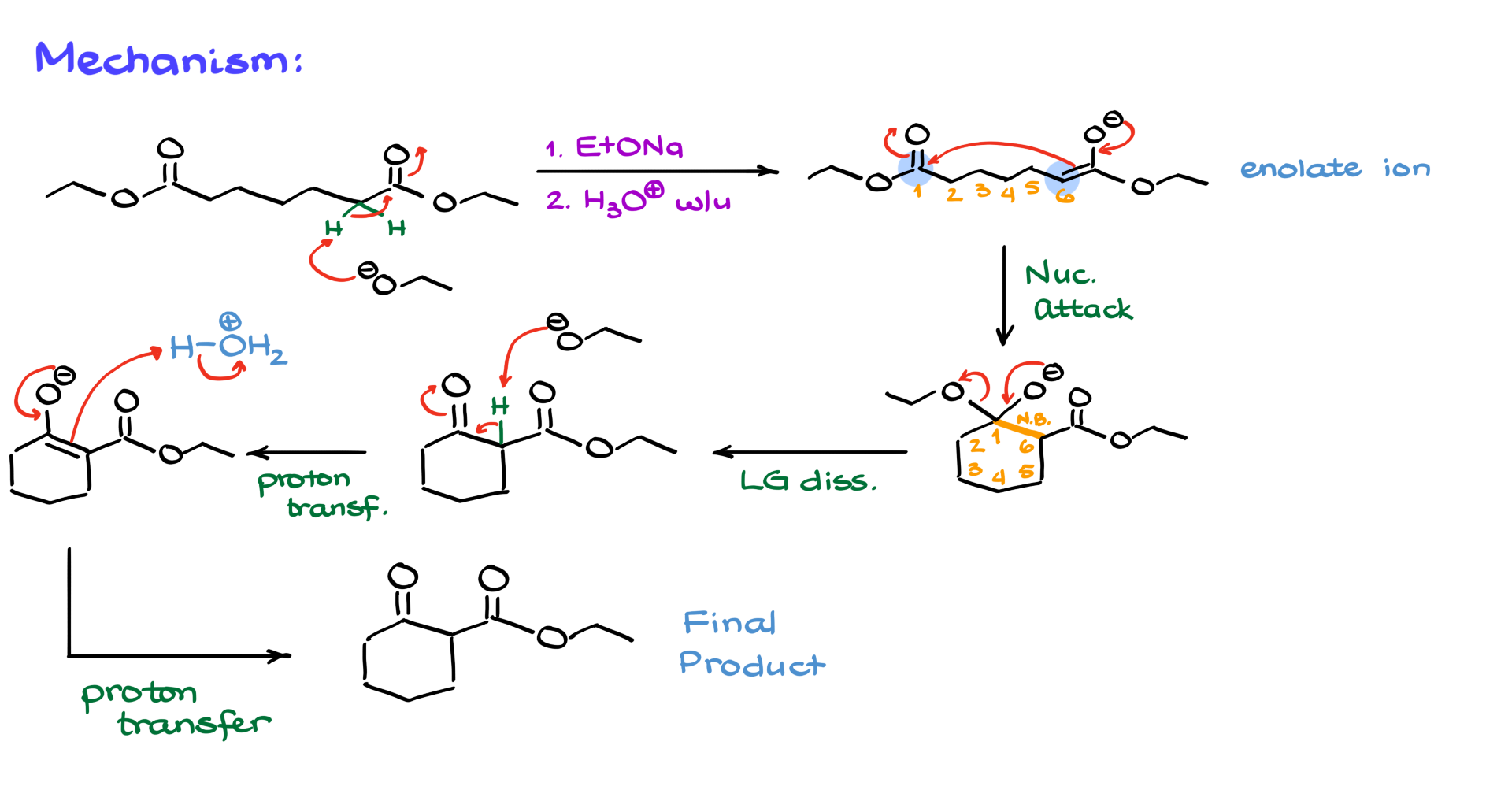
It doesn’t matter which alpha position is deprotonated in this case because I’ve chosen a completely symmetrical molecule. So, whether we deprotonate the left or right side, the result is the same. After this proton transfer, we get an enolate. Unlike the Claisen condensation, where we need a second equivalent of a carbonyl compound, here both the nucleophile (our enolate) and the electrophile (our carbonyl) are part of the same molecule, allowing them to react immediately.
When they do, the electron cascade forms a new carbon-carbon bond between the alpha carbon of the enolate and the carbon of the carbonyl, resulting in a six-membered ring. Drawing this cyclic product can be tricky, but here’s a helpful approach. First, number your carbons from the nucleophile to the electrophile or vice versa—either way works since these are just anchors. Knowing that the product is a six-membered ring, start by drawing the ring and numbering the atoms, either clockwise or counterclockwise. The numbering helps trace where each group and atom will be in the final product, making visualization easier. Highlight the new bond between carbons 1 and 6, and remember that carbon 1 bears a negatively charged oxygen and an ethoxy group. Carbons 2, 3, 4, and 5 have no substituents in this case, while carbon 6 holds the remaining part of the ester.
This intermediate is our tetrahedral intermediate, similar to what we see in the Claisen condensation. The next step involves the leaving group dissociating as the negatively charged oxygen kicks out the ethoxide, forming the dicarbonyl. But the mechanism doesn’t end there because the hydrogen between the carbonyls is relatively acidic, and the ethoxide formed immediately deprotonates that position, generating the corresponding enolate. The final step is the acidic workup, where water protonates the enolate to give the final product. Just like in the Claisen condensation, you’ll draw the same molecule twice—once as an intermediate that can’t be isolated because it’s immediately deprotonated, and once as the final product after protonation. Remember this step during exams, as the acidic workup is essential in both Claisen and Dieckmann condensations.
Dieckmann Condensation Example
Now, let’s consider a more challenging example where I’ve added an extra ethyl group at the alpha position, making the molecule no longer symmetrical.
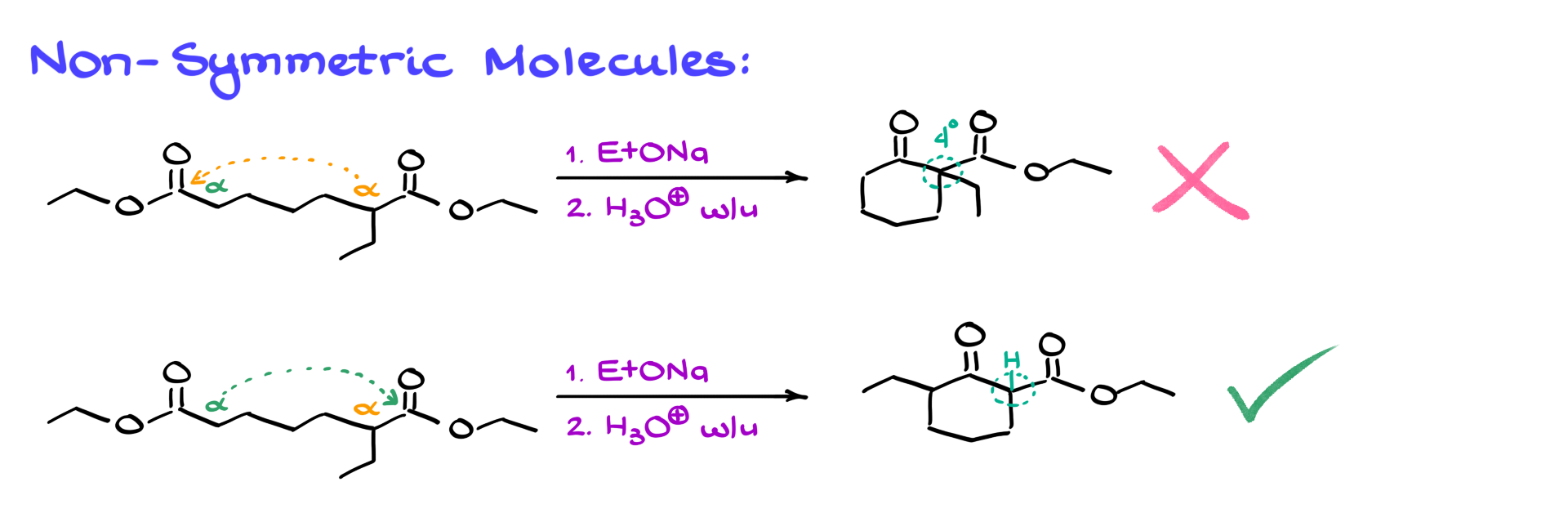
We have two different alpha positions—orange on the right and green on the left—leading to two potential products. One product forms when the orange alpha position is deprotonated and reacts with the left-side carbonyl, while the other forms when the green alpha position is deprotonated and reacts with the right-side carbonyl. While I’m using a shortcut to draw the final products here, make sure you can draw the full mechanism for both reactions. One of these products will be the major product, and the other will be a minor product. Here’s a hint: the key lies in the enolizable proton. Without enolization, the reaction can reverse easily, reopening the ring and returning to the starting material. Once that proton is removed, there’s no turning back. Thus, the product with the enolizable position is the major product, while the other, which would have a quaternary carbon between the carbonyls, will not form in any significant amount. In both Claisen and Dieckmann condensations, having an enolizable proton between the carbonyls is essential.
Another Example
Although there’s no true “mixed” or “crossed” Dieckmann condensation, we can have a Dieckmann-like reaction where one side of the molecule is a ketone and the other side is an ester.
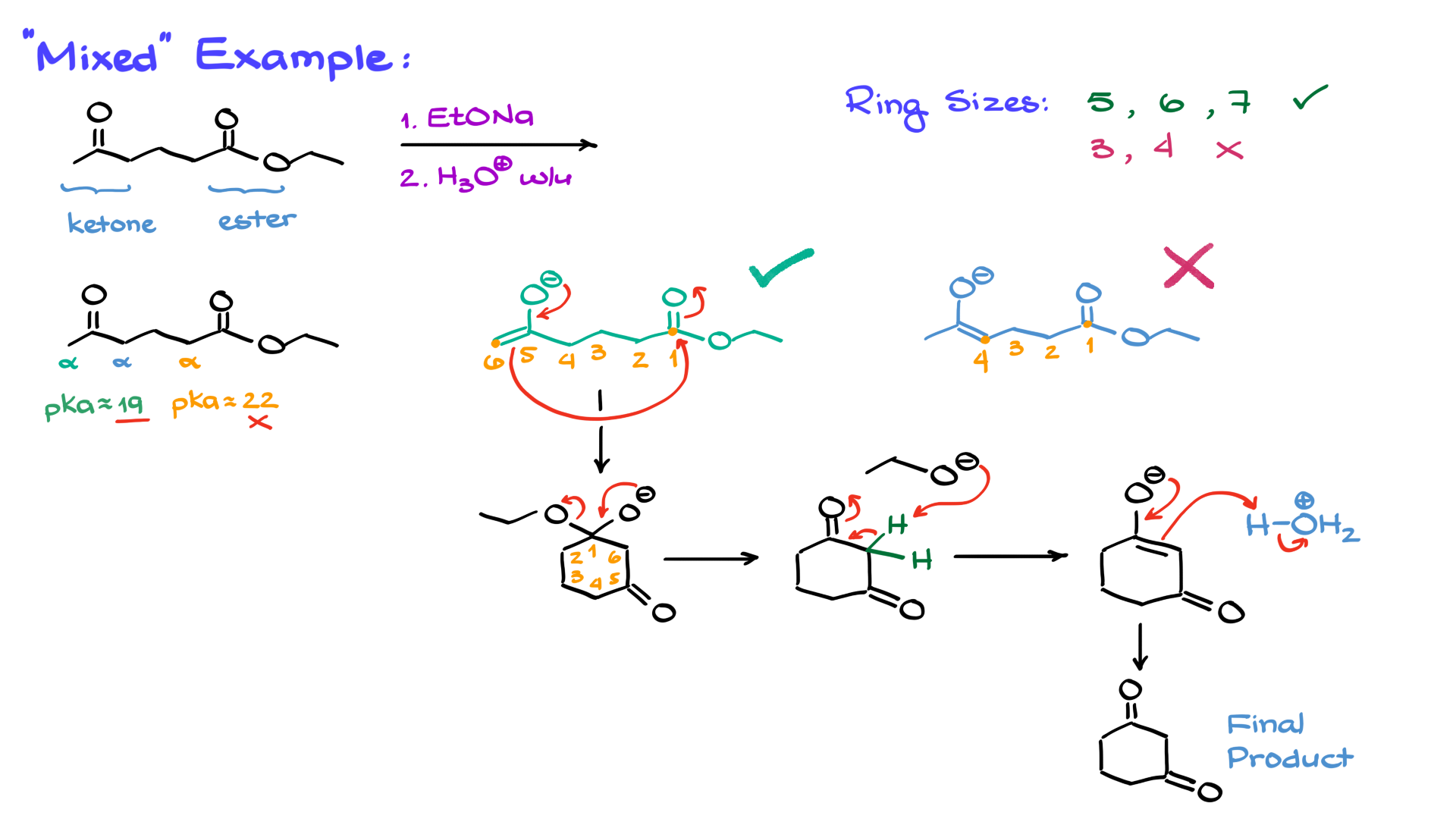
In such cases, the alpha positions have different acidities. For example, the alpha positions of a ketone are more acidic (with a pKa around 19) compared to the alpha position of an ester (pKa around 22-24). This significant difference means we prioritize deprotonating the more acidic position, effectively ignoring the less acidic alpha position. With two possible enolates, the choice depends on the size of the ring formed. Rings with 5, 6, or 7 members are stable, while 3 or 4-membered rings are too strained and unstable to form. Thus, the enolate that forms a stable ring is the one that proceeds through the reaction.
After forming the enolate, the nucleophile attacks the electrophile, forming a six-membered ring with a negatively charged oxygen on carbon 1 and a carbonyl on carbon 5. As before, the leaving group is expelled, forming a 1,3-cyclohexanedione intermediate. The ethoxide formed then deprotonates the dione, and subsequent protonation yields the final product. This deprotonation and reprotonation cycle might feel like a repetitive journey, even giving Bilbo Baggins a run for his money, but that’s how the mechanism works, and that’s how we need to draw it.
The key takeaway is that the Dieckmann condensation is essentially the Claisen condensation, with the difference that both reacting groups are part of the same molecule, leading to a ring as the final product. What do you think about the Dieckmann condensation? Do you like this reaction? Let me know in the comments below!