Robinson Annulation
In this tutorial, I want to talk about the Robinson annulation, which might seem like an intimidating reaction, but in reality, it’s pretty straightforward. Let me show you.
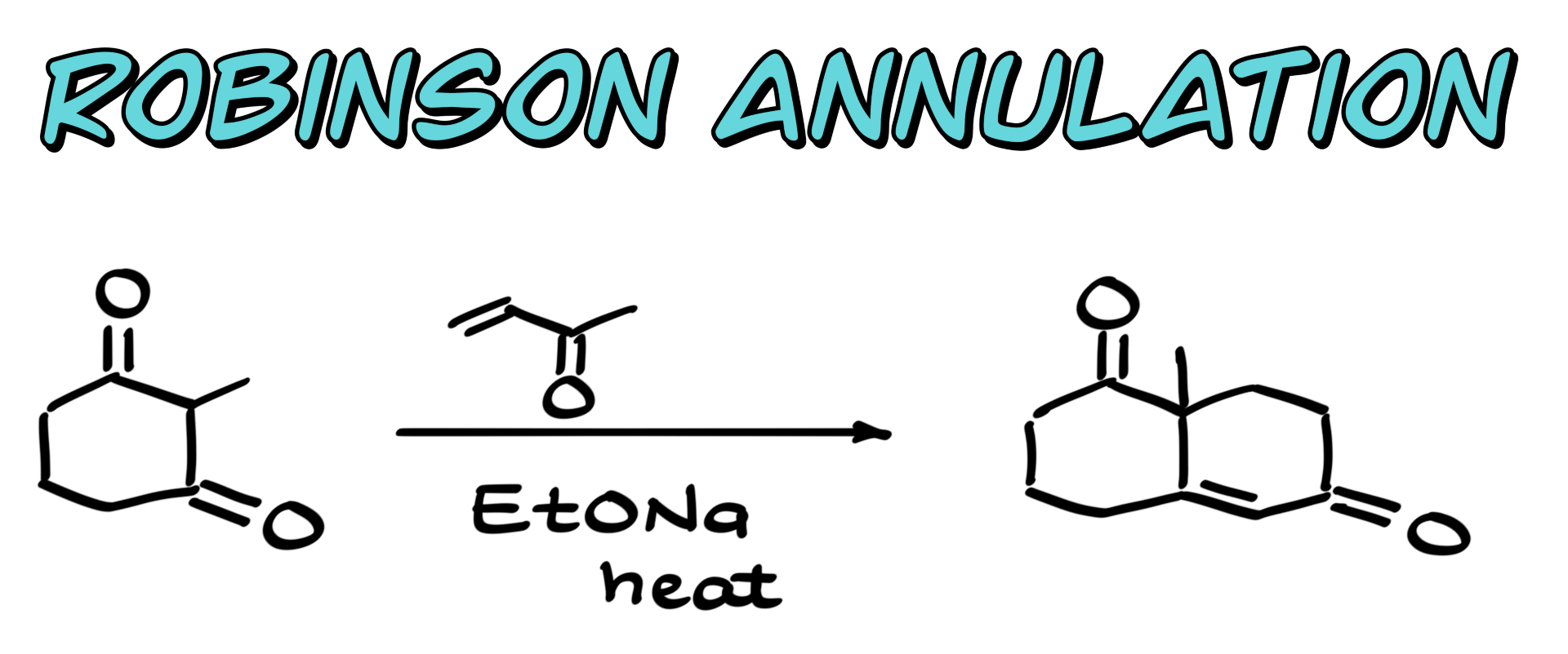
Robinson Annulation Mechanism
The idea is that we’re going to do a reaction between an enolizable carbonyl, like the one in the scheme below, which should ideally be a good Michael donor after enolization, and our second component, which will be an α,β-unsaturated carbonyl. The trick here is that we need to have an enolizable α-position in our α,β-unsaturated molecule. For instance, methylvinyl ketone (MVK) is the most common example of such a molecule. And of course, we need to use some base since we are dealing with enolate chemistry—sodium ethoxide is probably the most common go-to base you’ll see. Usually, we need a little bit of heat to push the reaction to completion.
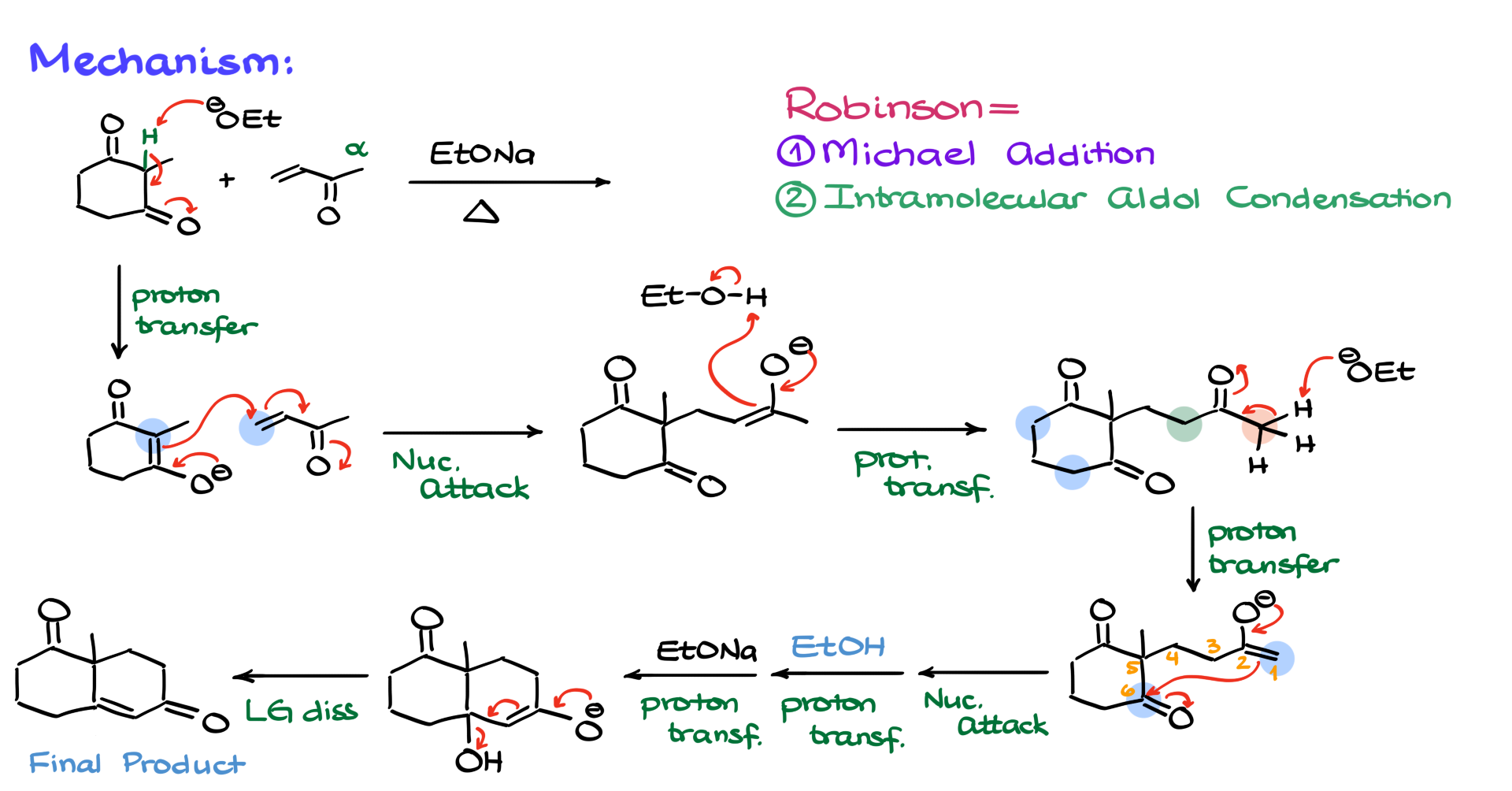
Now, the very first thing that’s going to happen in this mix of reagents is that the base, sodium ethoxide, will go after the most acidic proton. In our case, the most acidic proton is the one between the carbonyls. The pKa of this position is somewhere around 9, so sodium ethoxide will deprotonate it without any problems. This will generate the corresponding enolate. Now, this enolate is a perfect Michael donor, so we bring in our α,β-unsaturated compound, and a reaction occurs between them. The nucleophile, which is our enolate, performs a conjugate addition to the α,β-unsaturated compound, forming a new bond between the carbon adjacent to the carbonyl in our enolate and the β-position of the α,β-unsaturated compound. This gives us the enolate intermediate, which we can protonate with the ethanol that is floating around from our first step and is also our solvent, yielding the next carbonyl-containing intermediate.
Here’s something interesting about this carbonyl. We have multiple enolizable positions in this molecule. There are two identical blue positions, the green position (which we just had as an enolate), and an orange position at the end of the molecule. Since we are still in basic conditions, nothing stops us from re-enolizing this molecule at one of these positions. The green position doesn’t get us anywhere, but if we enolize at the orange position, we can do an intramolecular reaction by attacking one of the carbonyls. This will lead to a six-membered ring formation.
Let’s go through this mechanism properly. First, we remove a proton from the orange α-position using our base, forming the enolate. This enolate can now react with one of the carbonyls (it doesn’t matter which one, as they are identical), forming a new bond between this α-position and the carbon of the carbonyl. As I mentioned earlier, this will create a six-membered ring. Once this intramolecular nucleophilic attack occurs, we get a negatively charged intermediate, which we then protonate using the solvent. After this, the base re-enolizes the molecule, forming another enolate, and finally, we eliminate a leaving group, yielding the final product.
So, in a nutshell, the Robinson annulation is just a sequence of a Michael addition followed by an intramolecular aldol condensation. That’s it! As long as you follow these steps in order, you’ll always be able to get your product.
Robinson Annulation Example 1
Now, let’s look at an example.
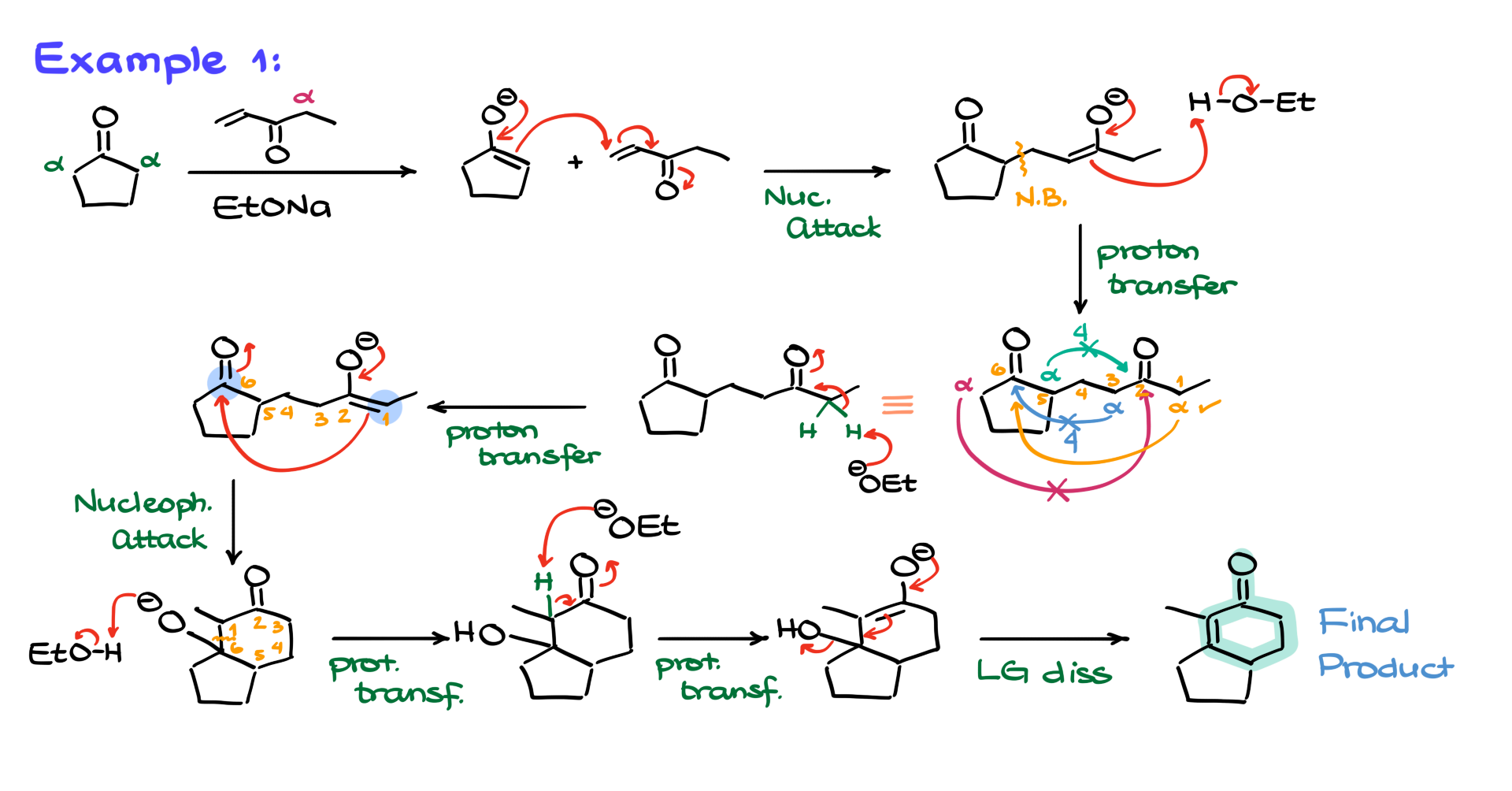
The first step is always to find the most acidic position among all the molecules. In this case, we have two identical green α-positions on our cyclopentanone, as well as an enolizable α-position on our α,β-unsaturated compound. Since α,β-unsaturated compounds are slightly less acidic in their α-positions than ketones, the green α-position is the most acidic and will be the target for enolization. The first step, then, is the formation of the enolate.
Next, we proceed with the Michael addition, where our nucleophile attacks the β-position of the α,β-unsaturated compound, forming a new bond at this position. This gives us an intermediate, which we then protonate using the solvent, yielding a neutral 1,5-dicarbonyl intermediate.
Now, we analyze the enolizable positions to determine the best route for the intramolecular aldol condensation. We have the pink α-position, the green α-position, the blue α-position, and the orange α-position. The pink α-position would lead to an awkwardly strained ring, so we rule that out. The green α-position would form a four-membered ring, which is also not ideal. The blue α-position would similarly result in a four-membered ring, making it a poor choice. Finally, the orange α-position, when reacting with the carbonyl, gives us a six-membered ring, which is the most favorable option.
So, we go with the orange α-position, enolizing it by removing a proton with our base to form the enolate. This enolate then attacks the carbonyl, forming a six-membered ring by creating a new bond between carbon 1 (our α-position) and carbon 6 (the carbonyl carbon). This leads to an intermediate where we protonate the negatively charged oxygen, yielding the aldol product. From here, we re-enolize the α-position, form another enolate, and eliminate a leaving group to obtain the final product.
One key thing to remember about the Robinson annulation is that in your final product, you will always see a six-membered ring with an α,β-unsaturated moiety. That is the signature of the Robinson annulation reaction. If your mechanism doesn’t yield that structural motif, you likely made a mistake somewhere. While the rest of the molecule can vary based on the starting materials, that specific structural feature will always be present.
Robinson Annulation Example 2
Alright, moving on—how about this example?
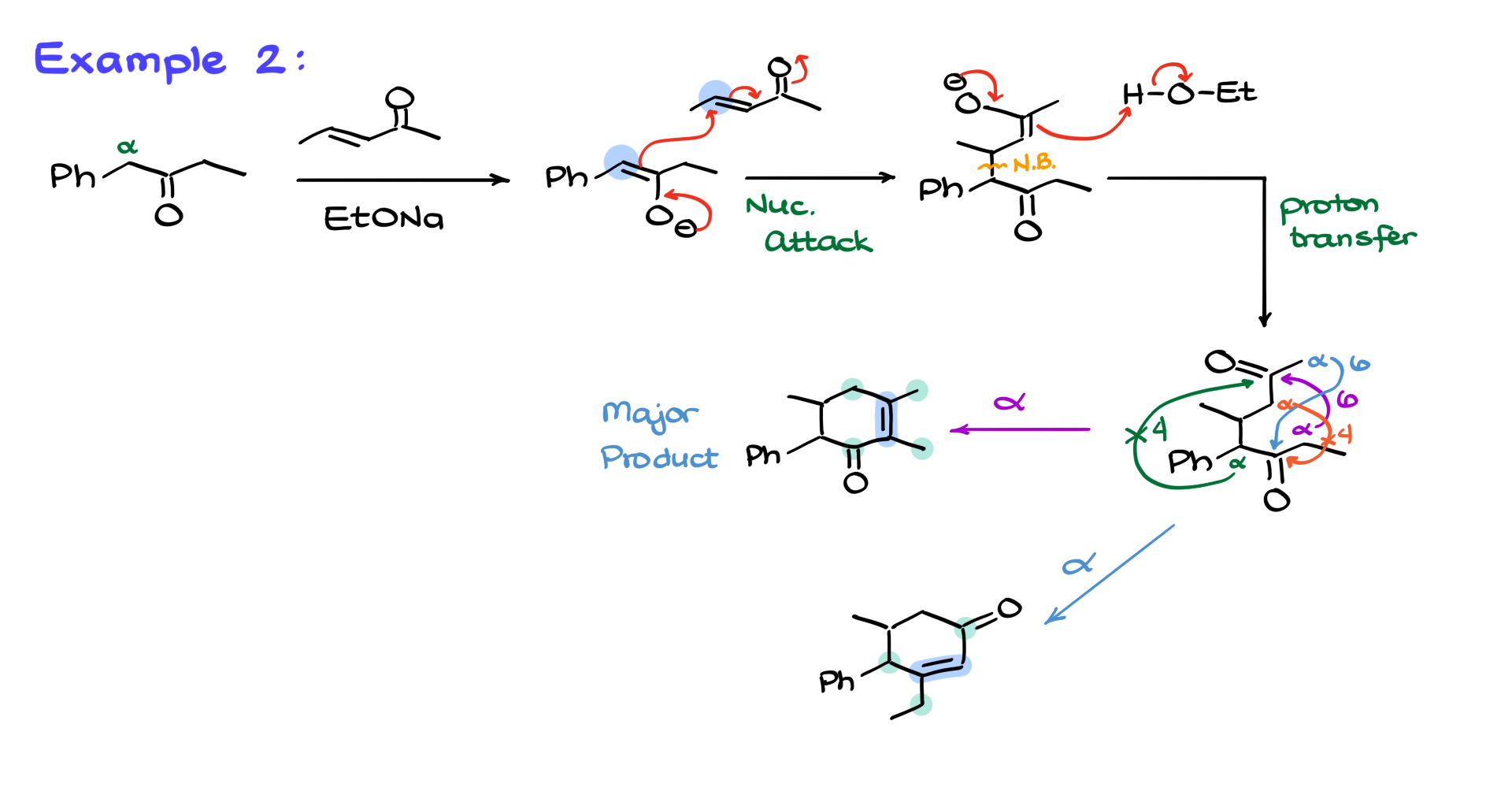
As usual, we start by identifying the most acidic position among our carbonyls. In this case, the best choice is the position between the carbonyl and the phenyl group since that enolate is additionally stabilized by resonance with the phenyl group, making it the easiest to form. So, we generate the enolate at this position and then bring in the α,β-unsaturated compound to start the Michael addition. The nucleophile attacks the β-position of the α,β-unsaturated compound, forming a new bond and giving us an enolate intermediate. We then protonate it, forming our 1,5-dicarbonyl.
Now, we analyze the enolizable positions to determine the best route for the intramolecular aldol condensation. We have the green, purple, orange, and blue α-positions. The green position, while the most acidic, would lead to a four-membered ring, which is highly unfavorable. The orange position also results in a four-membered ring, so we discard it as well. The purple and blue positions, however, both lead to six-membered rings, meaning we could form two possible products.
To determine which product is favored, we compare the stability of the double bonds in each case. The purple product has a fully substituted double bond with four substituents, whereas the blue product has a less substituted double bond with only three substituents. Since a more substituted double bond is generally more stable, the purple product is likely the major one.
Interestingly, I’ve seen this exact reaction appear in tests from two different instructors at two different schools, and they each gave different answers on which product was major. If it were up to me, I would say the purple product is the major one, but what do you think? Let me know in the comments below—are you team purple or team blue? Let’s get this discussion going!
Robinson Annulation Practice Questions
Would you like to see the answers and check your work?
Sign up or login if you’re already a member and unlock all members-only content!